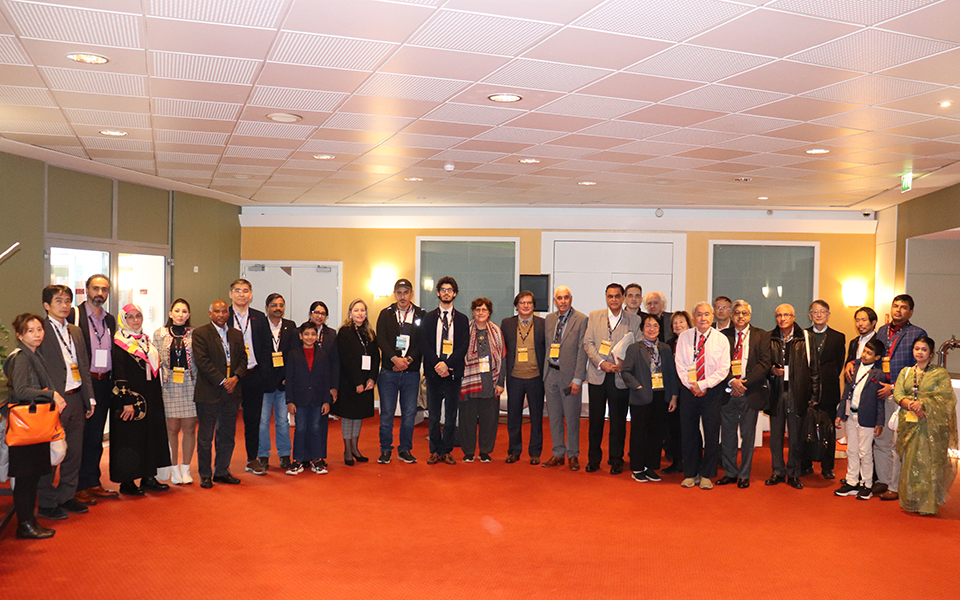
BioL-BioS 2023
Peers Alley Media,Canada
Primary goal of attending an international conference is to present a paper to the experts and influencers. It gives you a platform to exchange your interest-related thoughts, paving the way for possible future collaborations.
Use this platform to build connections with an elite group of wise men and women to enhance your intellect. Young entrepreneurs, this is a great platform to connect with your peers.
Knowledge is Power. Knowledge teaches skills. Skills define excellence. Use this platform to become cognizant of your interest area to achieve excellence in your domain.
Attending a conference give you opportunity to get your abstract or paper published in conference proceedings
Meet and greet a myriad of industry professionals and academia experts with common interest. Every meal will be an opportunity to meet and interact with fellow researchers, attendees and experts.
Expand your professional competency and learn useful tips and tricks of your industry in our skill-building workshops.
Explore insights on recent advancements, new equipment, new techniques, and unpublished data, learn from thought-leaders and get to network with a great line up of speakers.
Our exhibits floor offers the attendees with a dynamic display of the latest products with cutting-edge technology.
Investing in you is the best investment. Peers Alley conferences give the patrons with a feeling of the serendipity of real learning, skill development in strategic workshops, networking and start-up opportunities, thus, is value for money.
Attending the conference gives you much needed break from your regular duties. It also allows you to explore new cities, culture and meet new people. You will feel energized and rejuvenated to return to the university and continue with the job after attending the conference.
Conferences are vital forum for academic researchers and business leaders. "It involves multiple presentations, interactive breakout sessions, hands-on product demonstrations and unrivalled networking opportunities".
We have invited some of the world's most sought-after keynote speakers, experts, brand ambassadors, and industry leaders to share their thoughts and ideas with our conference guests.
Register Now
Adv.SEMS 2024 provides participants with a platform to explore cutting-edge research, collaborate with experts from various disciplines, and discover new opportunities for innovation and advancement in smart materials, energy materials and structures.
Here are 9 major themes that the congress could focus on:
• Smart Materials for Sustainable Infrastructure
• Bio-inspired Smart Materials and Biomimetics
• Smart Materials for Energy Harvesting and Storage
• Smart Materials for Healthcare and Biomedical Applications
• Multifunctional Smart Materials and Hybrid Systems
• Smart Materials for Urban Development and Smart Cities
• Smart Materials for Wearable Technology and Internet of Things
(IoT)
• Smart Materials for Advanced Manufacturing and Industry 4.0
• Smart Materials for Environmental Monitoring and Remediation
Adv. SEMS Proceedings
Dear Colleagues and Friends,
A warm welcome to the 7th Euro-Global Summit on Advancements in Smart Materials, Energy Materials, and Structures! We're thrilled to have you join us in Singapore on November 20-21, 2024.
Our goal for this gathering is simple: to bring together scientists, researchers, industry professionals, and academic scholars from all corners of the globe to explore the latest discoveries and innovations in materials science.
During the conference, we'll delve into key topics such as smart materials for sustainable infrastructure, bio-inspired smart materials, energy harvesting, healthcare applications, urban development, wearable technology, advanced manufacturing, and environmental monitoring.
Through engaging sessions, workshops, and presentations, we'll dive deep into the latest research and applications of smart materials. Our aim is to foster collaboration and accelerate innovation in this field, ultimately leading to sustainable solutions for global challenges.
The Advanced Smart Materials Market is on track to reach a remarkable $2.1 trillion by 2025, with a robust compound annual growth rate (CAGR) of 4.5% from 2020 to 2025. This surge is revolutionizing the global manufacturing sector and contributing to the escalating demand for advanced materials throughout the forecast period.
We're excited about the opportunity to learn from each other, exchange ideas, and forge new partnerships. Your participation is invaluable, and we look forward to a conference filled with insightful discussions and meaningful connections.
With best regards,
Adv. SEMS 2024
Organizing Committee
Peers Alley Media, Canada
University of Missouri–St. Louis, USA
II Professor, Ilinois Institute of Technology, USA
Chair Professor, West Virginia University, USA
Air Force Institute of Technology, USA
Active coatings for smart textiles refer to functional coatings that are applied to textile materials to add qualities like sensing, actuation, energy harvesting, and other responsive capabilities. These coatings allow textiles to interact with their environment, respond to stimuli, and perform functions beyond traditional textile materials.
Active coatings for smart textiles have been developing substantially in recent years, contributing to improvements in materials science, nanotechnology, and wearable technology.
Some significant advancements include:
Sensing Capabilities: Active coatings can include sensors that detect a variety of environmental parameters such as temperature, humidity, pressure, and even biological signs such as heart rate and movement. These sensors allow smart textiles to collect data and deliver significant insights for applications such as healthcare, sports, and monitoring systems.
Actuating Functions: Certain coatings can facilitate actuating functions, allowing smart textiles to respond to stimuli or perform mechanical actions. For example, shape memory polymers can be used in coatings to create shape-changing textiles, while piezoelectric materials can generate electrical energy from mechanical stress, enabling for energy harvesting or actuation.
Energy Harvesting: Active coatings may incorporate materials that harvest energy from their environment, such as solar radiation, body heat, or mechanical action. This harvested energy can power electronic components included in the textile or utilized as a backup power source for other devices.
Adaptive Properties: Advances in materials science have enabled the development of coatings with adaptive characteristics, such as self-healing capabilities that may repair damage or change properties in response to changing environment. These coatings improve the durability and usefulness of smart textiles, expanding their lifecycle and performance.
Integration with Electronics: Active coatings can help to integrate electronic components into textiles for applications such as flexible circuits, wearable displays, and communication systems. This integration allows for easy communication between the textile and external devices or networks.
Environmental Responsiveness: Some active coatings have been designed to respond to specific environmental stimuli, such as pH, temperature, or light exposure. These coatings can cause reversible changes in the fabric's qualities or appearance, allowing for dynamic camouflage, adaptive insulation, and responsive fashion designs.
Tags
Intelligent Materials Conferences
Smart Materials Conferences 2024 Japan
Carbon Materials Conferences
Nanotechnology Conferences 2024
Photovoltaic Materials Conferences
Composite Materials Conferences
Materials Science and Engineering Conferences
Advanced Materials Conferences
Nanotechnology Conferences 2024 USA
Piezoelectric Materials Conferences
Smart Materials Conferences 2024 Europe
Artificial Materials Conferences
Smart Materials Conferences 2024 Aisa
Materials Science Conferences 2024
Smart Materials Conferences 2024 USA
The term "adaptive materials" refers to substances that can modify their properties or behaviors in response to external stimuli. These materials are capable of adapting, changing, or reconfiguring themselves in response to certain stimuli such as temperature, light, pressure, or chemical changes. The advances in adaptable materials constitute a substantial leap in material science, with applications in a variety of industries.
Here are some major advancements in adaptable materials:
Shape Memory Alloys (SMAs): These materials can "remember" and return to a preset shape after being modified. These alloys are commonly utilized in applications requiring shape-changing characteristics, including as biomedical devices, actuators, and smart constructions.
Smart polymers: Smart polymers, also known as stimulus-responsive or shape-changing polymers, are characterized by reversible structural changes in response to external stimuli. For example; Hydrogels can alter shape and size in response to temperature, pH, and the presence of certain ions. These materials can be used in drugs delivery systems, sensors, and self-healing materials.
Photochromic and Thermochromic Materials: These materials that change color in response to changes in light (photochromic) or temperature (thermochromic). They are utilized in a range of applications, including smart fabrics, eyeglasses, and packaging, where color changes reflect temperature changes or light exposure.
Electroactive Polymers (EAPs): Electroactive polymers (EAPs) change shape or size in response to an electric field. This feature makes them ideal for use in robotics, artificial muscles, and haptic feedback systems.
Self-healing Materials: Self-healing Self-healing materials can autonomously repair damage, restoring structural integrity without the need for external intervention. This innovation has applications in automobile coatings, electronics, and construction materials, resulting in improved durability and endurance.
Magnetic Shape Memory Alloys (MSMAs): Magnetic Shape Memory Alloys (MSMAs) combine shape memory alloy characteristics with magnetic field response. These materials have uses in actuators, sensors, and medical devices.
Responsive Nanomaterials: Advances in nanotechnology have led to the development of nanomaterials having responsive features. Nanocomposites can respond to environmental stimuli in unique ways, providing improved performance in areas such as catalysis, drug delivery, and sensing.
Tags
Smart Materials Conferences 2024 Japan
Nanotechnology Conferences 2024
Nanotechnology Conferences 2024 Europe
Smart Materials Conferences 2024 Aisa
Nanotechnology Conferences 2024 Asia
Nanotechnology Conferences 2024 USA
Intelligent Materials Conferences
Smart Materials Conferences 2024 USA
Photovoltaic Materials Conferences
Composite Materials Conferences
Materials Science Conferences 2024 Europe
Artificial Materials Conferences
Smart Materials Conferences 2024
Advanced Materials Conferences
Materials Science Conferences 2024 USA
Advanced ceramics, also known as engineering ceramics or fine ceramics, are a type of high-performance material with better mechanical, thermal, electrical, and chemical capabilities than regular ceramics. These materials are designed for specific applications that require high performance in extreme conditions. Because of its distinct qualities and capabilities, modern ceramics have transformed a variety of industries.
Some important features of advanced ceramics and their emergence include:
Composition and Structure: Advanced ceramics are typically made up of non-metallic inorganic compounds like oxides, carbides, nitrides, and borides. They are frequently manufactured using precision methods such as sintering, hot pressing, or chemical vapor deposition to achieve high purity and homogeneous microstructures. For advanced ceramics to have the appropriate qualities, composition and structure must be carefully controlled.
Thermal Stability and Heat Resistance: Advanced ceramics are extremely stable when heated and heat resistant, allowing them to sustain high temperatures without distortion or degradation. They're utilized in high-temperature applications like thermal barriers, furnace linings, turbine components, and heat exchangers.
Electrical and electronic applications: Certain advanced ceramics have exceptional electrical qualities, such as high dielectric strength, low electrical conductivity, and good thermal conductivity. These characteristics make them suitable for use in electronic devices, semiconductor production, insulators, capacitors, and sensors.
Chemical Resistance: Advanced ceramics are frequently resistant to chemical corrosion and degradation, making them ideal for use in opposed chemical conditions. They find application in chemical processes, biomedical implants, corrosion-resistant coatings, and laboratory equipment.
Biocompatibility: Some modern ceramics, such as specific alumina and zirconia formulations, are biocompatible with living tissues, making them ideal for medical implants, dental restorations, and biomedical equipment.
Additive Manufacturing Emergence: Advanced ceramics have seen an increase in additive manufacturing, also known as 3D printing. Additive manufacturing enables the production of complex ceramic components with precise geometries and specific characteristics, creating new opportunities for design innovation and customisation across a wide range of sectors.
Overall, the rise of advanced ceramics has had a transformative impact on many industries, allowing for the creation of breakthrough products and technologies that push the limits of performance, efficiency, and dependability in demanding applications. Continued research and development in materials science are projected to improve the qualities and capabilities of advanced ceramics, propelling their use in even more diverse and challenging circumstances.
Tags
Smart Materials Conferences
Smart Materials Conferences 2024 Japan
Smart Materials Conferences 2024
Intelligent Materials Conferences
Composite Materials Conferences
Smart Materials Conferences 2024 Aisa
Materials Science and Engineering Conferences
Nanotechnology Conferences 2024
Materials Science Conferences 2024 Middle East
Materials Science Conferences 2024 Europe
Nanotechnology Conferences 2024 Asia
Nanotechnology Conferences 2024 USA
Smart Materials Conferences 2024 Europe
Materials Science Conferences 2024 Asia
Artificial Materials Conferences
Aircraft avionics, or aviation electronics, refers to the electronic systems used in aircraft for communication, navigation, monitoring, and control. Avionics are play a vital for assuring the safety, efficiency, and reliability of contemporary aircraft. Advances in airplane avionics have had a profound impact on both the aviation industry and the rest of the globe.
Here are some key aspects of aircraft avionics and their advancements:
Communication Systems: Advancements in avionics have resulted in more dependable and efficient communication systems, such as voice communication, data transmission, and satellite communication. Pilots can use these technologies to communicate with air traffic control, other aircraft, and ground stations, increasing airspace safety and coordination.
Navigation Systems: Advanced navigation technologies, such as GPS (Global Positioning System), have transformed airplane navigation by delivering accurate position information and route assistance to pilots. In addition to GPS, inertial navigation systems (INS) and ground-based navigation aids (such as VOR and ILS) are utilized to assure precise navigation under varying conditions.
Flight Management Systems (FMS): FMS combines navigation, flight planning, and performance monitoring functions into a single system, allowing pilots to manage flight operations more efficiently. These technologies improve aircraft safety and efficiency by automating various operations, optimizing flight paths, and providing real-time situational awareness.
Flight Control Systems: Advancements in avionics have resulted in the creation of fly-by-wire (FBW) and fly-by-light (FBL) flight control systems, which use electronic interfaces instead of traditional mechanical controls. These systems provide more precision, flexibility, and redundancy, enhancing aircraft handling and response.
Integrated Cockpit Displays: Modern aircraft have advanced cockpit displays, such as primary flight displays (PFDs) and multifunction displays (MFDs), that give pilots with extensive flight information, navigation data, weather updates, and system status indicators. These displays improve situational awareness and cockpit operations.
Health Monitoring Systems: Advances in avionics have enabled the development of health monitoring systems that continuously check aircraft systems and components for potential problems or anomalies. These devices provide early warning of possible failures, allowing for preventive maintenance and minimizing the chance of in-flight emergency.
Autonomous Systems: New technologies like artificial intelligence (AI) and autonomous flying systems are being integrated into aircraft avionics to automate specific activities, increase decision-making skills, and boost overall operating efficiency. These technologies have the potential to transform air travel by allowing autonomous aircraft operations and lowering reliance on human pilots.
Tags
Materials Science Conferences 2024 Middle East
Composite Materials Conferences
Materials Science Conferences 2024 USA
Materials Science Conferences 2024
Smart Materials Conferences 2024 Japan
Materials Science Conferences 2024 Asia
Nanotechnology Conferences 2024 Europe
Smart Materials Conferences
Materials Science Conferences
Smart Materials Conferences 2024 USA
Piezoelectric Materials Conferences
Nanotechnology Conferences 2024 Asia
Materials Science and Engineering Conferences
Nanotechnology Conferences 2024 Middle East
Artificial Materials Conferences
Artificial intelligence (AI) is a vast field in computer science focuses on creating machines or software that can perform tasks that typically require human intelligence. Learning, thinking, problem solving, natural language comprehension, speech recognition, and computer vision are some of these activities. AI is classified into two types: Narrow AI, which is developed for a single purpose, and General AI, which has the ability to understand, learn, and apply information across multiple areas, similar to human intelligence.
Advancements in Artificial Intelligence:
Machine Learning (ML): Machine Learning (ML) is a branch of artificial intelligence that involves the development of algorithms that enable computers to learn patterns and make decisions without explicit programming. Machine learning breakthroughs have led to improvements in predictive analytics, recommendation systems, and natural language processing.
Deep Learning: Deep learning is a type of machine learning, which involves neural networks with multiple layers (deep neural networks). This method has proven remarkable success in tasks which includes as the image and speech recognition, language translation, and gaming.
Natural Language Processing (NLP): NLP allows machines to understand, interpret, and generate human language. NLP breakthroughs have resulted in developments in chatbots, language translation, sentiment analysis, and virtual assistance.
Computer Vision: Artificial intelligence has made great progress in computer vision, allowing machines to analyze and make decisions based on visual data. This has uses in facial identification, object detection, autonomous vehicles, and medical image analysis.
Reinforcement Learning: This method involves instructing algorithms to make successive decisions by providing rewards or penalties. Reinforcement learning has shown effective in fields such as game playing, robotics, and optimization challenges.
Generative Adversarial Networks (GANs): Generative Adversarial Networks are made up of two neural networks, a generator and a discriminator, which are trained sequentially. They've been utilized to create realistic images, videos, and other multimedia.
Transfer Learning: Transfer learning enables models trained on one task to be used to a different but similar task. This has increased the effectiveness of training models and made AI applications more useful.
AI in Healthcare: AI breakthroughs have resulted in substantial developments in healthcare, such as diagnostic tools, customized medicine, drug discovery, and predictive analytics.
Quantum Computing: While still in its early stages, quantum computing has the potential to transform AI by greatly increasing its processing power and addressing complicated challenges more efficiently.
Tags
Photovoltaic Materials Conferences
Smart Materials Conferences
Smart Materials Conferences 2024 Europe
Materials Science Conferences 2024 Asia
Advanced Materials Conferences
Nanotechnology Conferences 2024 Middle East
Artificial Materials Conferences
Nanotechnology Conferences 2024 Asia
Materials Science Conferences 2024 Europe
Nanotechnology Conferences 2024 Europe
Nanotechnology Conferences 2024 USA
Materials Science Conferences 2024 USA
Smart Materials Conferences 2024 Aisa
Intelligent Materials Conferences
Materials Science Conferences 2024 Middle East
Biofunctionalized nanoparticles are nanoparticles that have been modified or coated with biological molecules to enhance their compatibility with living systems and enable specific interactions with biological entities. These nanoparticles have applications in various kinds of fields, including medicine, diagnostics, and environmental monitoring.
Advancements in Biofunctionalized Nanoparticles:
Drug Delivery: Biofunctionalized nanoparticles are designed for targeted drug delivery, which improves the efficiency and reducing side effects of medications by selectively delivering drugs to specific cells or tissues.
Imaging: They are used in biomedical imaging to improve contrast and visibility of tissues or specific biomolecules. Biofunctionalization enables the accurate targeting of imaging agents to biological structures.
Therapeutics: Biofunctionalized nanoparticles are used in therapeutic applications such as photothermal therapy and gene therapy that specifically target and treat diseased cells.
Diagnostic Tools: These nanoparticles are used as components of diagnostic tools to identify biomarkers, pathogens, and particular molecules that indicates the disease.
Biosensors: Biofunctionalized nanoparticles are integrated into biosensor platforms to detect biological analytes quickly and sensitively, contributing to advances in point-of-care diagnostics.
Cancer Treatment: Biofunctionalized nanoparticles are used in oncology to identify cancer early, deliver targeted drugs, and perform imaging for precise diagnosis and treatment monitoring.
Gene Editing: They are being explored in gene editing technologies to facilitate the delivery of gene-editing tools to specific cells or tissues for use in gene therapy.
Vaccine Development: Biofunctionalized nanoparticles are utilized in vaccine development to enhance the delivery of antigens and induce a more robust immune response.
Environmental Remediation: In environmental science, biofunctionalized nanoparticles are used to detect and remove pollutants, hence advancing environmental monitoring and remediation.
Neuroscience Applications: Biofunctionalized nanoparticles are being researched for their potential applications in neuroscience, such as targeted drug delivery to the brain and neural structure imaging.
Tags
Materials Science Conferences
Smart Materials Conferences 2024 Aisa
Materials Science Conferences 2024 USA
Smart Materials Conferences 2024 USA
Materials Science Conferences 2024 Europe
Nanotechnology Conferences 2024 Asia
Advanced Materials Conferences
Smart Materials Conferences
Materials Science Conferences 2024 Asia
Carbon Materials Conferences
Materials Science and Engineering Conferences
Nanotechnology Conferences 2024
Intelligent Materials Conferences
Composite Materials Conferences
Photovoltaic Materials Conferences
Bioinspired materials, also known as biomimetic materials, are substances that are inspired from the structures, properties, and processes observed in the natural world. Scientists and engineers design these materials to replicate or mimic certain characteristics of biological systems, such as tissue hierarchies, organisms' self-healing abilities, or the adhesive properties of particular surfaces.
Emergence and significance:
Innovation in Design: Bioinspired materials have emerged as a source of innovative design strategies for a variety of applications. Researchers developed the functionality, durability, and adaptability of materials by learning from the nature.
Medical Applications: Biomimetic materials have found use in medicine, including in the development of biocompatible implants, tissue engineering scaffolds, and drug delivery systems. These materials seek to replicate the structural and functional properties of natural tissues, hence improving compatibility with the human body.
Self-Healing Materials: The emergence of self-healing materials, inspired by living organisms' regenerative abilities, represents a significant achievement. These materials can repair damage themselves, leading to enhanced durability and lifespan in a variety of applications, including coatings and structural materials.
Adhesive Materials: Bioinspired adhesives were developed by examining the adhesion mechanisms of specific animals and insects. These adhesives have applications across industries, from healthcare (surgical adhesives) to manufacturing (joining materials without the use of mechanical fasteners).
Lightweight and Strong Materials: Researchers have successfully developed bioinspired materials that combine strength and low weight by emulating the structures of natural materials such as spider silk and bones, which are both lightweight and robust. The automotive and aerospace industries have affected by this.
Water-repellent Surfaces: Surfaces designed to repel water have been created, getting inspiration from the hydrophobic properties of the lotus leaf. These materials are utilized in the development of anti-fogging technologies, self-cleaning surfaces, and water-resistant compounds.
Energy Harvesting: Bioinspired materials have contributed to enhance energy harvesting systems. For example, researchers have studied photosynthesis to develop artificial systems for efficient solar energy conversion.
Environmental Sustainability: Scientists are currently developing materials and processes that are more environmentally sustainable by taking inspiration from the efficiency of nature. This includes researching methods for reducing manufacturing processes' pollution, energy consumption, and environmental impact.
Agriculture & Crop Protection: Biomimetic materials are also being explored for agricultural uses, such as the development of coatings that mimic the properties of plant surfaces to improve pest resistance or water retention.
Tags
Nanotechnology Conferences 2024 Europe
Materials Science Conferences 2024 Middle East
Materials Science Conferences 2024 Asia
Smart Materials Conferences 2024
Carbon Materials Conferences
Nanotechnology Conferences 2024 USA
Materials Science and Engineering Conferences
Smart Materials Conferences
Photovoltaic Materials Conferences
Materials Science Conferences 2024 Europe
Smart Materials Conferences 2024 Aisa
Composite Materials Conferences
Nanotechnology Conferences 2024 Asia
Biomimetic materials, also known as biomimicry or bio-inspired materials, are substances that replicate or inspired by biological processes, structures, and functions found in nature. The goal is to reproduce or imitate these natural designs in order to build materials with superior characteristics, performance, and functionality. This discipline has arisen as a result of advances in materials science, nanotechnology, and a better understanding of biological systems.
Key features of biomimetic materials are:
Structure and Design Inspiration: Biomimetic materials frequently draw inspiration from the hierarchical and complicated structures seen in living organisms, such as bones, shells, and plant fibers. Materials designed to mimic spider silk's tensile and lightweight properties, as well as the self-cleaning capabilities of lotus leaves, are two examples.
Functionality: Biomimetic materials aim to replicate specific functionalities found in nature, such as adhesion, self-healing, and environmental response. For example, materials inspired by gecko feet have been developed for their capacity of adhering to a variety of surfaces.
Applications: Biomimetic materials are finding applications in various industries, including medical, robotics, aircraft, and architecture. In medicine, scientists are exploring biomimetic materials for tissue engineering, drug delivery, and medical implants. In robotics, materials inspired by animal movements and structure help to develop more efficient and adaptive robotics systems.
Sustainability: Biomimetic materials frequently emphasize sustainability by replicating natural processes that are resource-efficient and environmentally favorable. For example, mimicking photosynthesis has influenced the development of solar energy conversion technologies.
Emerging Technologies: The emergence of biomimetic materials has resulted in the development of innovative technologies such as self-healing materials, bio-inspired sensors, and adaptive materials that respond to changes in their environment.
Challenges: Despite advancements, challenges remain in fully replicating complex biological systems and integrating biomimetic materials into practical applications.
Biomimicry research requires interdisciplinary collaboration among biologists, engineers, chemists, and material scientists.
Tags
Materials Science Conferences 2024 USA
Materials Science Conferences 2024 Asia
Composite Materials Conferences
Materials Science and Engineering Conferences
Smart Materials Conferences 2024 USA
Nanotechnology Conferences 2024 Asia
Photovoltaic Materials Conferences
Nanotechnology Conferences 2024
Carbon Materials Conferences
Nanotechnology Conferences 2024 Europe
Nanotechnology Conferences 2024 Middle East
Piezoelectric Materials Conferences
Smart Materials Conferences 2024 Europe
Smart Materials Conferences 2024 Japan
Intelligent Materials Conferences
"Chromo" typically relates to color, and "active" suggests a dynamic or responsive nature. As a result, chromoactive materials could be materials that exhibit color-changing or responsive properties.
Such materials have several potential advancements and applications:
Smart Displays: Chromoactive materials could be utilized to develop displays that change color or appearance in response to user activities, ambient conditions, or specific signals. This could result in more dynamic and adaptive user interfaces.
Sensors and Indicators: These materials could be used as sensors or indicators. For example, in environmental monitoring, they may change color in the presence of specific pollutants or poisons, offering a visual indication of environmental conditions.
Health Monitoring: Chromoactive materials combined into wearable devices or medical sensors have the potential to function as indicators of a wide range of health parameters. A color-changing patch, for example, may convey signal changes in body temperature or blood sugar levels.
Security Features: Chromoactive materials could be used to prevent anti-counterfeiting measures, such as currency or product packaging that changes color in reaction to certain situations. This could help improve security and authentication procedures.
Aesthetics and Design: In terms of design and aesthetics, chromoactive materials could be used to produce dynamic and visually appealing products from clothes to architectural features that change color dependent on environmental circumstances or customer preferences.
Responsive Materials: Chromoactive materials have the potential to help produce responsive materials in a variety of industries. For example, in construction, materials that change color in response to stress or structural concerns may provide early notice of future problems.
Tags
Piezoelectric Materials Conferences
Nanotechnology Conferences 2024
Intelligent Materials Conferences
Nanotechnology Conferences 2024 Middle East
Smart Materials Conferences 2024 Aisa
Materials Science Conferences 2024 Europe
Materials Science Conferences 2024 USA
Nanotechnology Conferences 2024 Europe
Smart Materials Conferences 2024 Europe
Photovoltaic Materials Conferences
Smart Materials Conferences
Materials Science Conferences 2024 Middle East
Smart Materials Conferences 2024 Japan
Carbon Materials Conferences
Smart Materials Conferences 2024
Composite materials are engineered materials composed of two or more constituent materials having distinctly different physical or chemical properties. These materials are mixed to form a new material with increased properties not found in any of the constituent components alone. The elements typically consist of a reinforcing material (commonly fibers or particles) and a matrix substance that holds the reinforcements together.
Key types of composite materials are:
Fiber-Reinforced Composites: These composites are consist of high-strength fibers (such as carbon, glass, or aramid fibers) inserted in a matrix (usually polymer, metal, or ceramic). Examples include carbon fiber-reinforced polymers (CFRP) are commonly utilized in aircraft, automobile, and sports equipment.
Particulate Composites: Particles (such as ceramics, metals, or polymers) are dispersed within a matrix material. Composite materials with metal particles are used to improve conductivity, whereas ceramics are used to increase strength.
Laminar Composites: Layers of different materials accumulate together, with each layer possessing unique features. Common examples of laminar composites include plywood and laminated glass.
Structural Composites: Composites utilized in load-bearing applications, such as construction materials or bridge and building components.
Advancements and Contributions of Composite Materials:
Lightweight and strong: One of the main benefits of composite materials is their high strength-to-weight ratio. This feature is especially beneficial in the aircraft, automotive, and sports industries, where lightweight but strong materials are critical for fuel efficiency, performance, and durability.
Corrosion Resistance: Composite materials are frequently resistant to corrosion, making them suitable for use in unfriendly environments. This is particularly helpful for marine and offshore structures.
Tailored Properties: Engineers can vary this type of orientation, and volume fraction of the reinforcing materials to tailor the properties of composite materials to meet specific performance requirements.
Design Flexibility: Composite materials offer design flexibility, allow for the creation of complicated shapes and structures that would be challenging with traditional materials.
Durability and Longevity: Many composites are extremely durable and fatigue resistant, allowing materials to last longer and require less maintenance.
Thermal and electrical conductivity: Composite materials can be designed to have specific thermal and electrical conductivity properties, making them suitable for a wide range of applications such as electronics and thermal management systems.
Energy Efficiency: The usage of composite materials in transportation helps to enhance energy efficiency by reducing vehicle weight, which leads to better fuel economy.
Green and Sustainable Materials: Advances in composite material technology are also helping to develop environmentally friendly and sustainable materials which incorporate bio-based reinforcements and recyclable matrices.
Tags
Materials Science Conferences 2024 Asia
Smart Materials Conferences 2024 Europe
Nanotechnology Conferences 2024
Materials Science Conferences
Smart Materials Conferences 2024 Aisa
Smart Materials Conferences 2024
Advanced Materials Conferences
Piezoelectric Materials Conferences
Materials Science and Engineering Conferences
Carbon Materials Conferences
Materials Science Conferences 2024 Middle East
Nanotechnology Conferences 2024 Middle East
Materials Science Conferences 2024 Europe
Nanotechnology Conferences 2024 Asia
Nanotechnology Conferences 2024 Europe
Conductive polymers are a type of material that exhibits electrical conductivity, similar to traditional metals, but are made up of long-chain macromolecules. Unlike normal insulators, conductive polymers have conjugated electron systems that allow electrical charges to pass through them. This unique combination of properties makes them adaptable materials with applications in several kinds of fields.
Here are some key aspects and potential breakthroughs:
Basic Properties: Conductive polymers usually have a π-electron system along the polymer backbone, which enables the passage of charge carriers (electrons or holes) and provides electrical conductivity. They can be made to be p-type (positive charge carriers) or n-type (negative charge carriers) by modifying their chemical structures.
Applications:
Flexible Electronics: Conductive polymers are utilized in flexible and stretchable electronics due to their inherent flexibility.
Sensors: They find application among sensors that detect various chemical and physical parameters.
Anti-static Coatings: Conductive polymers are used to form anti-static coatings on materials, which reduce the buildup of static electricity.
Advancements and breakthroughs:
Organic Electronics: Conductive polymers have provided significant contributions to the field of organic electronics, including the development of organic light-emitting diodes (OLEDs), organic solar cells, and organic field-effect transistors. These applications are crucial to the development of lightweight, flexible, and low-cost electronic devices.
Energy Storage Devices: Conductive polymers have been explored for use in energy storage devices such as batteries and super capacitors. They have the potential for higher specific capacitance and energy density.
Biomedical Applications: Significant advances have been made in the use of conductive polymers for biomedical applications. Because of their compatibility with biological systems, they have been explored for use in neural interfaces, biosensors, and drug delivery systems.
Conductive Hydrogels: The invention of conductive hydrogels based on polymers has opened up new avenues for soft robotics, wearable electronics, and bioelectronics.
Tags
Smart Materials Conferences 2024 Japan
Smart Materials Conferences
Materials Science Conferences
Artificial Materials Conferences
Advanced Materials Conferences
Nanotechnology Conferences 2024 USA
Materials Science Conferences 2024 Europe
Photovoltaic Materials Conferences
Composite Materials Conferences
Materials Science Conferences 2024 USA
Nanotechnology Conferences 2024 Asia
Materials Science Conferences 2024 Asia
Smart Materials Conferences 2024 USA
Nanotechnology Conferences 2024 Europe
Smart Materials Conferences 2024
Elastomers are polymers with unique properties that give them high elasticity and the ability to return to their original shape after being stretched or deformed. They are widely used in various industries due to their flexibility, resilience, and insulating properties.
The advancements in elastomers have had a significant impact on different aspects of the world:
Automotive Industry: Elastomers, particularly rubber compounds, are extensively used in the automotive industry for tires, gaskets, seals, hoses, and suspension components. Advances in elastomer technology contribute to the development of more durable and fuel-efficient tires, reducing overall environmental impact.
Consumer Goods: Elastomers are found in a myriad of consumer products, including footwear, clothing, kitchenware, and various molded goods. Advanced elastomers enhance the comfort, durability, and performance of consumer goods.
Medical Devices: In the medical field, elastomers are crucial for applications such as medical tubing, gloves, seals, and other devices due to their biocompatibility and flexibility. Advancements in medical-grade elastomers contribute to the development of safer and more reliable medical devices.
Electronics and Electrical Applications: Elastomers serve as insulating materials in electronics, providing electrical insulation and protection. Advancements in elastomeric materials contribute to the development of more reliable and durable electronic components, especially in harsh environments.
Smart Materials: Researchers are exploring the development of smart elastomers that respond to external stimuli, such as changes in temperature, pressure, or electrical fields. Smart elastomers have potential applications in sensors, actuators, and adaptive materials, expanding the range of possibilities for responsive and intelligent systems.
High-Performance Elastomers: Advances in polymer chemistry and material science have led to the creation of high-performance elastomers with improved mechanical properties, chemical resistance, and durability. These high-performance elastomers find applications in demanding environments, such as aerospace, where reliability and performance under extreme conditions are crucial.
Sustainability: There is a growing emphasis on developing sustainable elastomers, including bio-based and recycled materials, to reduce the environmental impact of these polymers. Sustainable elastomers contribute to eco-friendly manufacturing processes and end-of-life disposal considerations.
Tags
Carbon Materials Conferences
Materials Science Conferences
Piezoelectric Materials Conferences
Smart Materials Conferences
Composite Materials Conferences
Photovoltaic Materials Conferences
Materials Science Conferences 2024 Europe
Smart Materials Conferences 2024 Japan
Intelligent Materials Conferences
Nanotechnology Conferences 2024
Smart Materials Conferences 2024
Nanotechnology Conferences 2024 USA
Materials Science Conferences 2024
Materials Science Conferences 2024 Asia
Electroactive polymers (EAPs) are a class of polymers that exhibit significant and reversible deformation in response to an electric field. These materials are capable of undergoing large deformations when subjected to electrical stimulation and can revert to their original shape when the electric field is removed. Electroactive polymers have gained attention for their potential use in various applications, and advancements in this field have opened up new possibilities. Here are key aspects and advancements in electroactive polymers:
Types of Electroactive Polymers:
Dielectric Polymers: These polymers deform due to the electrostatic forces induced by the application of an electric field. Common examples include silicone elastomers and acrylic elastomers.
Conductive Polymers: These polymers can undergo changes in conductivity in response to an applied voltage. Examples include polypyrrole, polyaniline, and polythiophene.
Advancements and Applications:
Actuators and Artificial Muscles: One of the primary applications of electroactive polymers is in the development of actuators and artificial muscles. These materials can mimic the movement of natural muscles and find applications in robotics, prosthetics, and haptic devices.
Sensors: Electroactive polymers are used as sensors to detect changes in mechanical strain, pressure, or touch. They are employed in applications such as force sensors, tactile displays, and medical sensors.
Energy Harvesting: EAPs have been explored for energy harvesting applications, where mechanical deformations induced by ambient vibrations or movements are converted into electrical energy.
Soft Robotics: The field of soft robotics benefits from electroactive polymers due to their ability to deform and actuate in response to electrical stimuli. Soft robotic devices are being developed for applications in healthcare, exploration, and human-machine interaction.
Biomedical Devices: Electroactive polymers are investigated for use in biomedical devices, including drug delivery systems and tissue engineering scaffolds. Their biocompatibility and ability to mimic biological movements make them suitable for various medical applications.
Responsive Materials: Advancements have been made in designing electroactive polymers with specific responsiveness to different stimuli, such as light or temperature changes, expanding their range of applications.
Challenges and Future Directions: Challenges in the field include improving the mechanical properties, durability, and response times of electroactive polymers. Ongoing research is focused on developing new materials and improving the understanding of the underlying mechanisms to enhance the performance and reliability of electroactive polymers.
Tags
Composite Materials Conferences
Artificial Materials Conferences
Carbon Materials Conferences
Materials Science and Engineering Conferences
Smart Materials Conferences 2024 Japan
Nanotechnology Conferences 2024 USA
Materials Science Conferences 2024 Middle East
Materials Science Conferences 2024 Europe
Smart Materials Conferences 2024
Materials Science Conferences 2024 Asia
Smart Materials Conferences 2024 Aisa
Nanotechnology Conferences 2024
Nanotechnology Conferences 2024 Middle East
Nanotechnology Conferences 2024 Asia
Advanced Materials Conferences
Electro-rheological fluids (ERFs) are a type of smart fluid that changes viscosity or flow behavior in response to an electric field. When an electric field is applied, the particles or molecules suspended in the fluid align themselves in a specific manner, causing the fluid to become more viscous or even solidify. Conversely, when the electric field is removed, the fluid returns to its original state, typically within milliseconds.
Here's an overview of electro-rheological fluids and their breakthroughs:
Basic Principle: Electro-rheological fluids consist of suspended particles dispersed in a carrier fluid, often oil or silicone oil.
The particles typically have a polar nature and can align themselves in response to an electric field due to electrostatic forces.
As the particles align, they create chains or structures that impede the flow of the fluid, resulting in an increase in viscosity or the formation of a solid-like state.
Applications:
Smart Dampers and Shock Absorbers: ERFs are used in automotive and aerospace industries for adaptive suspension systems that can adjust damping characteristics in real-time based on road conditions or vehicle dynamics.
Vibration Control: ERFs find applications in vibration control systems for buildings, bridges, and machinery to mitigate vibrations and enhance structural stability.
Clutches and Brakes: ERFs are utilized in clutches and brakes to provide precise and rapid control of torque transmission or braking force.
Haptic Feedback: ERFs are employed in haptic feedback devices to simulate different tactile sensations by varying the viscosity of the fluid in response to user interactions.
Tunable Mechanical Properties: ERFs enable the development of materials with tunable mechanical properties, such as stiffness and damping, which find applications in robotics, prosthetics, and active damping systems.
Improved Performance: Advances in particle synthesis and fluid formulation have led to ERFs with enhanced stability, response time, and tunability of rheological properties.
Miniaturization: Breakthroughs in microfluidics and nanotechnology have enabled the development of miniaturized ERF-based devices suitable for microscale applications, such as microfluidic systems and lab-on-a-chip devices.
Multifunctionality: Researchers are exploring ERFs with multifunctional capabilities, such as simultaneous control of mechanical, thermal, and optical properties, opening up new possibilities for integrated smart materials and devices.
Novel Applications: Ongoing research is uncovering novel applications of ERFs in fields such as soft robotics, wearable technology, and biomedical devices, where precise control of mechanical properties is critical.
Tags
Smart Materials Conferences 2024 USA
Smart Materials Conferences
Materials Science and Engineering Conferences
Piezoelectric Materials Conferences
Intelligent Materials Conferences
Nanotechnology Conferences 2024 Europe
Smart Materials Conferences 2024 Japan
Materials Science Conferences 2024 USA
Photovoltaic Materials Conferences
Composite Materials Conferences
Nanotechnology Conferences 2024 Asia
Materials Science Conferences 2024
Materials Science Conferences 2024 Asia
Nanotechnology Conferences 2024
Artificial Materials Conferences
Energy harvesting refers to the process of capturing and converting ambient forms of energy, such as light, heat, vibration, or motion, into electrical energy for use in electronic devices. The goal is to harness energy from the surrounding environment to power low-energy electronic systems or recharge batteries. Advancements in energy harvesting technologies have been significant, leading to more efficient and versatile systems.
Here are key aspects and breakthroughs in energy harvesting:
Types of Energy Harvesting:
Photovoltaic Harvesting: Photovoltaic cells convert light energy into electrical energy. Advances in solar cell technology have improved efficiency and reduced the cost of photovoltaic energy harvesting.
Thermoelectric Harvesting: This method captures energy from temperature differences. Advances in thermoelectric materials have enhanced the efficiency of converting heat gradients into electricity.
Vibration Harvesting: Devices such as piezoelectric materials can convert mechanical vibrations or movements into electrical energy. Miniaturized and more sensitive materials contribute to better vibration harvesting systems.
RF (Radio Frequency) Harvesting: Harvesting ambient RF signals, like those from Wi-Fi or radio signals, has seen advancements, enabling the wireless powering of low-energy devices.
Applications:
Wireless Sensor Networks: Energy harvesting is crucial for powering wireless sensors in remote or inaccessible locations, eliminating the need for frequent battery replacements.
Wearable Electronics: Advances in flexible and lightweight energy harvesters contribute to the development of self-powered wearable devices, reducing reliance on traditional batteries.
Internet of Things (IoT): Energy harvesting is vital for many IoT applications, enabling sensors and devices to operate efficiently in various environments.
Medical Devices: Implantable medical devices benefit from energy harvesting, allowing for extended device lifetimes and reduced invasive procedures for battery replacements.
Remote and Autonomous Systems: Energy harvesting is employed in systems where frequent battery replacement or external power sources are impractical, such as in remote monitoring equipment or environmental sensors.
Breakthroughs and Advancements:
Miniaturization: Progress in micro and nanotechnology has allowed for the miniaturization of energy harvesting devices, making them suitable for integration into small and portable electronics.
Improved Efficiency: Ongoing research focuses on enhancing the efficiency of energy harvesting systems, optimizing the conversion of ambient energy into usable electrical power.
Multi-Modal Harvesting: Combining multiple energy harvesting techniques (e.g., solar and vibration harvesting) in a single device allows for more comprehensive energy capture in diverse environments.
Energy Storage Solutions: Advancements in energy storage technologies, such as more efficient and long-lasting batteries or supercapacitors, complement energy harvesting systems, ensuring a stable power supply during periods of low ambient energy availability.
Tags
Artificial Materials Conferences
Materials Science Conferences 2024 USA
Composite Materials Conferences
Photovoltaic Materials Conferences
Materials Science Conferences 2024
Smart Materials Conferences 2024 Aisa
Nanotechnology Conferences 2024
Nanotechnology Conferences 2024 Middle East
Piezoelectric Materials Conferences
Advanced Materials Conferences
Nanotechnology Conferences 2024 Asia
Materials Science Conferences 2024 Asia
Materials Science Conferences
Materials Science and Engineering Conferences
Smart Materials Conferences
Flexible electronics and wearable sensors represent a burgeoning field at the intersection of electronics, materials science, and biomedical engineering. These technologies involve the development of electronic devices and sensors that are lightweight, bendable, stretchable, and conformable to various surfaces, including human skin. They offer a wide range of potential applications across multiple sectors, including healthcare, fitness, sports, military, fashion, and more.
Here are some key advancements and their implications:
Healthcare Monitoring: Wearable sensors enable continuous monitoring of vital signs such as heart rate, blood pressure, body temperature, and respiratory rate. This real-time data can be invaluable for early detection and management of health conditions, remote patient monitoring, and improving overall healthcare outcomes.
Fitness and Sports: Athletes and fitness enthusiasts can benefit from wearable sensors that track performance metrics like activity levels, calorie expenditure, muscle activity, and hydration status. These devices provide valuable insights for optimizing training routines, preventing injuries, and enhancing athletic performance.
Remote Patient Monitoring: Flexible electronics allow for the development of wearable medical devices that can remotely monitor patients with chronic conditions, elderly individuals, or post-surgery patients. This technology enables healthcare providers to remotely track patients' health parameters, detect abnormalities, and intervene when necessary, thereby reducing hospitalizations and healthcare costs.
Prosthetics and Rehabilitation: Flexible sensors integrated into prosthetic limbs and orthopedic devices can provide feedback on movement, pressure, and muscle activity, improving the functionality and comfort of these devices. Additionally, wearable sensors can assist in rehabilitation by monitoring movements during physical therapy sessions and providing feedback to patients and therapists.
Smart Textiles and Fashion: Advancements in flexible electronics have led to the development of smart textiles embedded with sensors and actuators. These textiles can monitor physiological parameters, adjust to environmental conditions (e.g., temperature, humidity), and even change color or shape based on user preferences or external stimuli, opening up new possibilities for interactive clothing and fashion.
Military and Defense: Wearable sensors play a crucial role in military applications for monitoring soldiers' health and performance in the field. These devices can track vital signs, detect environmental hazards, and provide situational awareness, enhancing soldier safety and mission effectiveness.
Environmental Monitoring: Flexible sensors can be deployed in environmental monitoring systems to measure air quality, water pollution, and other environmental parameters. These sensors enable continuous, real-time data collection in various locations, helping to assess environmental health, detect pollution sources, and inform decision-making for resource management and conservation efforts.
Tags
Materials Science Conferences 2024 Middle East
Materials Science Conferences 2024 Europe
Materials Science Conferences 2024
Artificial Materials Conferences
Carbon Materials Conferences
Nanotechnology Conferences 2024 Europe
Materials Science Conferences 2024 Asia
Smart Materials Conferences 2024 Japan
Nanotechnology Conferences 2024 Middle East
Composite Materials Conferences
Advanced Materials Conferences
Photovoltaic Materials Conferences
Materials Science and Engineering Conferences
Nanotechnology Conferences 2024
Smart Materials Conferences
The functionalization of carbon nanotubes (CNTs) involves modifying their surface properties and structure to tailor their behavior for specific applications. Carbon nanotubes are cylindrical nanostructures composed of carbon atoms arranged in a hexagonal lattice, and functionalization enhances their compatibility with various materials and improves their performance in a wide range of applications.
Here are some key aspects of CNT functionalization and its advancements for the world:
Enhanced Mechanical and Electrical Properties: Functionalization can improve the dispersion of carbon nanotubes in various matrices, such as polymers or composites. This leads to enhanced mechanical and electrical properties of the resulting materials. Reinforcing polymers with functionalized CNTs can result in lightweight, strong, and conductive materials with applications in aerospace, automotive, and structural engineering.
Biomedical Applications: Functionalized carbon nanotubes show promise in biomedical applications. They can be tailored to interact with biological molecules and tissues, enabling applications such as drug delivery, imaging, and biosensing. Functionalized CNTs can serve as carriers for targeted drug delivery systems, improving the efficiency and specificity of treatments.
Sensors and Detectors: Functionalized carbon nanotubes have been employed in the development of highly sensitive sensors and detectors. Their high surface area and unique electronic properties make them suitable for detecting gases, chemicals, and biomolecules. This has applications in environmental monitoring, healthcare diagnostics, and security systems.
Energy Storage and Conversion: Functionalized CNTs play a role in improving the performance of energy storage devices such as batteries and supercapacitors. They can be used as electrodes to enhance conductivity and capacitance, contributing to the development of more efficient and high-performance energy storage systems.
Catalysis and Nanotechnology: Functionalized carbon nanotubes can serve as catalyst supports, promoting various catalytic reactions. This has implications for industrial processes, including the production of chemicals and fuels. Additionally, functionalized CNTs are being explored in nanotechnology applications, such as the development of nanoelectronics and nanocomposites.
Water Purification: Functionalized carbon nanotubes have demonstrated effectiveness in water purification and treatment processes. They can be functionalized to selectively adsorb and remove contaminants, such as heavy metals and organic pollutants, from water sources. This has the potential to address water pollution challenges globally.
Flexible Electronics and Wearables: Functionalized carbon nanotubes find applications in flexible electronics and wearable devices. They can be integrated into flexible substrates, contributing to the development of lightweight and bendable electronic components. This is particularly relevant for advancements in wearable technology and flexible displays.
Thermal Management: Functionalized carbon nanotubes exhibit excellent thermal conductivity. As a result, they are used in thermal interface materials for electronic devices, contributing to improved heat dissipation and thermal management. This is crucial for maintaining the performance and reliability of electronic components.
Tags
Materials Science Conferences 2024
Smart Materials Conferences
Photovoltaic Materials Conferences
Smart Materials Conferences 2024 USA
Materials Science and Engineering Conferences
Nanotechnology Conferences 2024 Europe
Composite Materials Conferences
Smart Materials Conferences 2024
Nanotechnology Conferences 2024 USA
Nanotechnology Conferences 2024 Middle East
Intelligent Materials Conferences
Advanced Materials Conferences
Materials Science Conferences
Materials Science Conferences 2024 USA
Smart Materials Conferences 2024 Aisa
Graphene-based materials are a class of materials that incorporate graphene, a single layer of carbon atoms arranged in a two-dimensional honeycomb lattice. Graphene exhibits remarkable properties, including exceptional electrical conductivity, mechanical strength, flexibility, and thermal conductivity. These properties make graphene and graphene-based materials highly attractive for various applications across multiple industries.
Some notable breakthroughs and advancements in graphene-based materials include:
Electronic Devices: Graphene's extraordinary electrical conductivity has led to breakthroughs in the development of electronic devices. Graphene transistors have demonstrated high-speed operation and low power consumption, promising advancements in high-performance electronics, flexible displays, and wearable technology. Additionally, graphene-based materials are being explored for applications in sensors, photodetectors, and transparent conductive films.
Energy Storage: Graphene-based materials hold promise for next-generation energy storage devices, such as batteries and supercapacitors. Graphene's high surface area, mechanical strength, and electrical conductivity enhance the performance of electrodes in these devices, enabling faster charging rates, higher energy density, and longer cycle life. Breakthroughs in graphene-based batteries and supercapacitors could revolutionize the energy storage landscape, facilitating the adoption of renewable energy sources and electric vehicles.
Membranes and Filtration: Graphene-based membranes have shown promise for various filtration and separation applications. Graphene's impermeability to gases and liquids, combined with its nanoscale pores, enables the selective passage of molecules and ions. Breakthroughs in graphene membranes have implications for water purification, desalination, gas separation, and drug delivery, offering more efficient and sustainable solutions for addressing environmental and healthcare challenges.
Biomedical Applications: Graphene-based materials are being explored for a range of biomedical applications, including drug delivery, tissue engineering, biosensing, and imaging. Graphene's biocompatibility, large surface area, and tunable properties make it an attractive platform for targeted drug delivery systems, biosensors, and bioimaging agents. Breakthroughs in graphene-based biomedical technologies could lead to advancements in personalized medicine, diagnostics, and regenerative medicine.
Flexible Electronics and Wearables: Graphene's mechanical flexibility and electrical conductivity make it well-suited for flexible electronics and wearable devices. Graphene-based flexible displays, sensors, and wearable health monitors have the potential to revolutionize consumer electronics, healthcare, and human-computer interaction. Breakthroughs in graphene-based flexible electronics enable the development of lightweight, conformable, and energy-efficient devices with enhanced functionality and performance.
Thermal Management: Graphene's exceptional thermal conductivity makes it an attractive material for thermal management applications. Graphene-based materials are being investigated for heat dissipation in electronic devices, thermal interface materials, and thermal energy harvesting. Breakthroughs in graphene-based thermal management technologies could improve the efficiency and reliability of electronic systems while enabling new opportunities in energy conversion and storage.
Tags
Advanced Materials Conferences
Nanotechnology Conferences 2024 Middle East
Materials Science Conferences 2024
Smart Materials Conferences 2024
Smart Materials Conferences 2024 Europe
Smart Materials Conferences
Nanotechnology Conferences 2024 Europe
Nanotechnology Conferences 2024 Asia
Artificial Materials Conferences
Materials Science Conferences 2024 Europe
Photovoltaic Materials Conferences
Intelligent Materials Conferences
Materials Science and Engineering Conferences
Materials Science Conferences 2024 USA
Materials Science Conferences 2024 Asia
Hydrogel-based bioinks are a critical component in the field of 3D bioprinting, a technology that allows the fabrication of three-dimensional biological structures layer by layer. These bioinks serve as the printable materials that encapsulate living cells and provide a supportive environment for their growth and organization. Hydrogels are water-absorbing, cross-linked polymers that have a high water content, similar to the natural extracellular matrix (ECM) found in tissues.
Advancements in hydrogel-based bioinks have been crucial for improving the accuracy, viability, and functionality of 3D bioprinted constructs. Here are some key aspects of hydrogel-based bioinks and their advancements:
Biocompatibility: Hydrogels used as bioinks need to be biocompatible to ensure that they support cell viability and function. Advances in hydrogel chemistry have led to the development of bioinks with improved biocompatibility, closely mimicking the native tissue microenvironment.
Printability: The rheological properties of hydrogel-based bioinks play a significant role in the printing process. Researchers have focused on optimizing these properties to achieve better printability, allowing for the precise deposition of bioink layers and the creation of complex structures.
Cell Encapsulation: Hydrogel-based bioinks are designed to encapsulate living cells, protecting them during the printing process and providing a conducive environment for their proliferation and differentiation. Advances in bioink formulations aim to enhance cell viability and maintain cell functionality post-printing.
Mechanical Properties: Mimicking the mechanical properties of native tissues is essential for the successful application of 3D bioprinted constructs. Researchers have worked on adjusting the mechanical properties of hydrogel-based bioinks to match those of specific tissues, promoting proper tissue integration and functionality.
Biochemical Signals: Hydrogel bioinks can be modified to incorporate biochemical cues such as growth factors, peptides, and other signaling molecules. This allows researchers to create bioinks that not only provide physical support but also guide cell behavior and tissue development.
Multi-material Printing: Advancements in 3D bioprinting technology have enabled the simultaneous use of multiple bioinks with different properties. This allows for the creation of heterogeneous structures with varying cell types, hydrogel compositions, and mechanical characteristics within a single construct.
Vascularization: Achieving vascularization in 3D bioprinted tissues is crucial for their survival and integration into host tissues. Hydrogel-based bioinks have been modified to facilitate the development of vascular networks within the printed constructs, promoting nutrient and oxygen supply to encapsulated cells.
In Vivo Applications: Researchers are exploring the potential of hydrogel-based bioinks for in vivo applications, aiming to use 3D bioprinting to create functional tissues and organs for transplantation or regenerative medicine.
Tags
Nanotechnology Conferences 2024 USA
Materials Science Conferences 2024 USA
Smart Materials Conferences 2024 Aisa
Smart Materials Conferences 2024
Materials Science and Engineering Conferences
Materials Science Conferences
Smart Materials Conferences 2024 Europe
Materials Science Conferences 2024 Asia
Carbon Materials Conferences
Smart Materials Conferences 2024 Japan
Piezoelectric Materials Conferences
Materials Science Conferences 2024
Smart Materials Conferences
Smart Materials Conferences 2024 USA
Nanotechnology Conferences 2024 Middle East
Intelligent packaging materials refer to packaging technologies that incorporate functionalities beyond traditional passive containment and protection. These materials are designed to interact with the packaged product, the environment, or external stimuli to provide additional benefits such as monitoring, extending shelf life, ensuring product authenticity, and enhancing user experience. Advancements in intelligent packaging materials have been driven by innovations in materials science, nanotechnology, sensors, and digital technologies. Some key advancements include:
Smart Sensors: Integration of sensors into packaging materials allows real-time monitoring of various parameters such as temperature, humidity, gas composition, and physical integrity. These sensors provide valuable data throughout the supply chain to ensure product quality and safety.
Active Packaging: Active packaging materials incorporate substances or systems that actively interact with the packaged product to extend shelf life or maintain product freshness. Examples include oxygen scavengers, moisture absorbers, antimicrobial agents, and ethylene absorbers.
Nanotechnology Integration: Nanomaterials offer unique properties such as enhanced barrier properties, improved mechanical strength, and responsiveness to external stimuli. Intelligent packaging materials leveraging nanotechnology provide better protection against oxygen, moisture, and microbial contamination while enabling novel functionalities.
QR Codes and RFID Tags: Integration of QR (Quick Response) codes and RFID (Radio-Frequency Identification) tags into packaging materials enables product traceability, authentication, and supply chain visibility. Consumers can access product information, authentication features, and interactive content by scanning these codes with their smartphones.
Blockchain Technology: Blockchain technology provides a secure and transparent way to record and track product-related information across the supply chain. Intelligent packaging materials integrated with blockchain enable immutable records of product provenance, quality, and authenticity, thereby enhancing trust and transparency.
Responsive Materials: Responsive packaging materials change their properties in response to external stimuli such as temperature, light, moisture, or pressure. These materials can provide indicators of product freshness, tampering, or exposure to adverse conditions, enhancing consumer safety and confidence.
Biodegradable and Sustainable Materials: Advancements in sustainable packaging materials include the development of biodegradable polymers, compostable films, and renewable resources. Intelligent packaging solutions that prioritize environmental sustainability aim to minimize waste and reduce the environmental footprint of packaging materials.
Tags
Materials Science Conferences 2024 Asia
Carbon Materials Conferences
Smart Materials Conferences 2024 USA
Smart Materials Conferences 2024 Japan
Piezoelectric Materials Conferences
Nanotechnology Conferences 2024 Asia
Intelligent Materials Conferences
Smart Materials Conferences 2024 Aisa
Materials Science Conferences
Nanotechnology Conferences 2024
Materials Science Conferences 2024 Europe
Artificial Materials Conferences
Materials Science Conferences 2024
Magnetic nanomaterials are materials at the nanoscale (typically ranging from 1 to 100 nanometers) that exhibit magnetic properties. These materials are composed of magnetic elements or compounds, such as iron, cobalt, nickel, or their alloys, structured in nanosized configurations. Magnetic nanomaterials have garnered significant interest due to their unique magnetic properties and potential applications in various fields, including medicine, electronics, environmental remediation, and data storage.
Biomedical Applications:
Drug Delivery: Magnetic nanoparticles can be functionalized with drugs or therapeutic agents and guided to specific target sites within the body using an external magnetic field, enabling targeted drug delivery with reduced side effects.
Hyperthermia Therapy: Magnetic nanoparticles can generate heat when exposed to an alternating magnetic field. This property is exploited in hyperthermia therapy for cancer treatment, where localized heating of tumor cells using magnetic nanoparticles helps in tumor ablation.
Magnetic Resonance Imaging (MRI): Magnetic nanomaterials with tailored magnetic properties are used as contrast agents in MRI to enhance image resolution and improve the detection of diseased tissues.
Data Storage: Breakthroughs in magnetic nanomaterials have contributed to the development of high-density magnetic storage media for data storage applications. Magnetic nanoparticles with stable magnetic properties enable the fabrication of smaller and more efficient magnetic storage devices with increased storage capacity.
Sensors and Electronics: Magnetic nanomaterials are integrated into sensors and electronic devices for various applications, including magnetic field sensors, magnetic random-access memory (MRAM), and spintronics. These materials enable the fabrication of smaller, faster, and more energy-efficient electronic devices.
Environmental Remediation: Magnetic nanomaterials are utilized in environmental remediation processes for the removal of contaminants from air, water, and soil. Functionalized magnetic nanoparticles can selectively bind to pollutants, facilitating their removal through magnetic separation techniques.
Catalysis: Magnetic nanomaterials serve as catalysts or catalyst supports in various chemical reactions due to their high surface area and magnetic properties. These materials enable efficient catalytic processes for applications such as hydrogen production, pollutant degradation, and chemical synthesis.
Energy Applications: Magnetic nanomaterials are explored for energy-related applications, including magnetic refrigeration, energy storage devices, and magnetic field-assisted water splitting for hydrogen production. These materials offer opportunities for improving energy efficiency and sustainability.
Tags
Smart Materials Conferences 2024
Smart Materials Conferences 2024 Aisa
Materials Science Conferences 2024 Middle East
Smart Materials Conferences
Smart Materials Conferences 2024 Japan
Materials Science Conferences 2024
Materials Science Conferences 2024 Asia
Materials Science Conferences 2024 Europe
Nanotechnology Conferences 2024
Nanotechnology Conferences 2024 Asia
Composite Materials Conferences
Nanotechnology Conferences 2024 Middle East
Smart Materials Conferences 2024 USA
Piezoelectric Materials Conferences
Materials Science and Engineering Conferences
Magnetic Shape Memory Alloys (MSMAs) are a class of smart materials that exhibit both shape memory effect and magnetic properties. These alloys can undergo reversible shape changes in response to an external magnetic field. The most common type of MSMA is based on the Ni-Mn-Ga system, where nickel is replaced partially by manganese and gallium. When exposed to a magnetic field, these alloys can change their shape and return to their original shape when the magnetic field is removed. This unique combination of properties makes MSMAs attractive for various applications.
Improved Magnetic Field Responsiveness: Researchers have been working on optimizing the composition and microstructure of MSMA to enhance their response to magnetic fields. This includes achieving higher magnetic field-induced strain and faster response times.
Increased Operating Temperatures: Early MSMA materials had limitations regarding their operating temperatures. Recent advancements involve the development of alloys with improved stability and functionality at higher temperatures, expanding their range of potential applications.
Miniaturization and Integration: Advancements in manufacturing techniques have enabled the production of MSMA components at smaller scales. This allows for the integration of MSMA elements into microelectromechanical systems (MEMS) and other miniaturized devices.
Biomedical Applications: MSMAs have shown promise in biomedical applications, such as minimally invasive surgeries and drug delivery systems. Researchers are exploring the use of MSMA-based actuators for precise control of medical devices within the body.
Energy Harvesting: MSMAs can be employed in energy harvesting devices, converting magnetic energy into mechanical energy. This application is being explored for scavenging energy from ambient magnetic fields to power small electronic devices.
Adaptive Structures and Morphing Systems: MSMA materials are used in the development of adaptive structures and morphing systems in aerospace and engineering applications. These materials enable the design of components that can change shape in response to varying conditions, improving overall system performance and efficiency.
Smart Materials in Robotics: The unique properties of MSMAs make them suitable for applications in robotics. Researchers are exploring the use of MSMA-based actuators and sensors in the development of responsive and adaptable robotic systems.
Enhanced Fatigue Resistance: Addressing the issue of fatigue is a key area of advancement. Researchers are working on improving the fatigue resistance of MSMAs, ensuring that they maintain their functionality over a more extended period of use.
Tags
Nanotechnology Conferences 2024 Europe
Smart Materials Conferences 2024 Europe
Nanotechnology Conferences 2024
Intelligent Materials Conferences
Composite Materials Conferences
Piezoelectric Materials Conferences
Materials Science Conferences 2024 Middle East
Smart Materials Conferences 2024 USA
Nanotechnology Conferences 2024 Asia
Smart Materials Conferences 2024 Japan
Materials Science Conferences
Artificial Materials Conferences
Smart Materials Conferences 2024 Aisa
Materials Science Conferences 2024 Europe
Carbon Materials Conferences
Magneto-rheological (MR) fluids are smart materials that can change their rheological (flow) properties in response to an external magnetic field. These fluids typically consist of small, suspended ferrous particles in a carrier liquid. When a magnetic field is applied, the alignment of these particles results in changes to the fluid's viscosity, stiffness, and other rheological properties. The ability to dynamically control these properties makes magneto-rheological fluids valuable in various engineering applications. Breakthroughs in this field have expanded their use in diverse sectors.
Vibration and Shock Damping: MR fluids are utilized in shock absorbers and dampers to control vibrations and impacts in vehicles, machinery, and structures. The ability to adjust the fluid's viscosity in real-time allows for adaptive damping, providing a smoother ride and improved vehicle or equipment performance.
Clutch and Braking Systems: MR fluids find application in clutches and braking systems where their rheological properties can be altered to control torque transmission or braking force. This allows for precise and rapid adjustments in response to changing conditions, enhancing vehicle safety and performance.
Robotics and Haptic Devices: In robotics and haptic technology, MR fluids are employed to create variable resistance or damping in joints and actuators. This enables the development of more responsive and adaptable robotic systems and enhances the realism of haptic feedback in virtual reality environments.
Seismic Dampers and Base Isolation Systems: MR fluids are incorporated into seismic dampers and base isolation systems for buildings and bridges. The ability to adjust the damping properties in real-time helps mitigate the impact of earthquakes by reducing structural vibrations and displacements.
Advanced Manufacturing: In certain manufacturing processes, MR fluids are used to create smart tools that can adapt their mechanical properties during machining or cutting operations. This helps optimize the manufacturing process and improve the quality of finished products.
Energy Dissipation Devices: MR fluids are employed in devices designed to dissipate excess mechanical energy. This includes applications in shock absorbers for vehicles, where the ability to adjust damping in response to varying road conditions enhances ride comfort and stability.
Tags
Materials Science Conferences
Smart Materials Conferences 2024 Japan
Materials Science and Engineering Conferences
Smart Materials Conferences 2024 Europe
Materials Science Conferences 2024
Materials Science Conferences 2024 Middle East
Materials Science Conferences 2024 USA
Smart Materials Conferences 2024 Aisa
Smart Materials Conferences
Nanotechnology Conferences 2024 Europe
Composite Materials Conferences
Smart Materials Conferences 2024 USA
Nanotechnology Conferences 2024
Nanotechnology Conferences 2024 Middle East
Metamaterials are engineered materials with properties not found in naturally occurring substances. They're designed to manipulate electromagnetic waves, such as light and sound, in ways that aren't possible with conventional materials. Metamaterials derive their properties from their precise structure rather than their composition alone.
Advancements in metamaterials have been significant and diverse:
Cloaking Devices: Metamaterials have been used to create cloaking devices that can hide objects from specific wavelengths of light or other electromagnetic waves. This concept has applications in military stealth technology, as well as in medical imaging and other fields.
Superlenses: Metamaterials have enabled the creation of superlenses capable of capturing images with resolutions beyond the diffraction limit of conventional optics. This has implications for microscopy and nanotechnology.
Acoustic Metamaterials: These are used to manipulate sound waves, allowing for the creation of sound barriers, acoustic cloaks, and devices that can control the direction of sound waves. Applications include noise reduction, ultrasound imaging, and architectural acoustics.
Optical Metamaterials: Metamaterials have been developed to manipulate light at the nanoscale, leading to innovations in optical devices such as lenses, waveguides, and filters. These materials have potential applications in telecommunications, sensors, and photovoltaics.
Tunable Metamaterials: Researchers have developed metamaterials whose properties can be dynamically adjusted, allowing for real-time control over their behavior. This capability opens up new possibilities in areas such as adaptive optics, reconfigurable antennas, and frequency-selective surfaces.
Energy Harvesting: Metamaterials have been explored for harvesting energy from various sources, including sunlight, thermal radiation, and mechanical vibrations. By efficiently capturing and converting energy, these materials have the potential to improve the performance of renewable energy systems and extend the lifespan of batteries.
Topological Metamaterials: Inspired by concepts from topology, researchers have developed metamaterials with exotic properties such as topological protection and robustness against defects. These materials may find applications in quantum computing, spintronics, and other areas of advanced electronics.
Tags
Carbon Materials Conferences
Materials Science Conferences 2024 Middle East
Materials Science Conferences 2024 USA
Smart Materials Conferences 2024 Aisa
Nanotechnology Conferences 2024 USA
Intelligent Materials Conferences
Materials Science Conferences 2024 Asia
Smart Materials Conferences 2024 USA
Nanotechnology Conferences 2024 Middle East
Materials Science Conferences 2024 Europe
Smart Materials Conferences
Materials Science Conferences
Nanotechnology Conferences 2024 Asia
Composite Materials Conferences
Microfluidic devices are miniaturized systems that manipulate small amounts of fluids (typically in the microliter to nanoliter range) within channels or chambers with dimensions on the microscale. These devices are designed to perform various tasks, such as chemical analysis, biochemical reactions, drug delivery, and diagnostics, in a controlled and precise manner. The field of microfluidics has witnessed several breakthroughs that have expanded its applications and capabilities:
Lab-on-a-Chip Technology: One of the key breakthroughs in microfluidics is the development of lab-on-a-chip (LOC) devices. These chips integrate multiple laboratory functions onto a single micro-sized platform. This has enabled high-throughput screening, point-of-care diagnostics, and automation of complex analytical processes.
Point-of-Care Diagnostics: Microfluidic devices have been instrumental in creating portable and low-cost diagnostic tools. These devices can be used for rapid and on-site detection of diseases, pathogens, and biomarkers. Breakthroughs in this area have led to the development of diagnostics for conditions like infectious diseases, cancer, and diabetes.
Organs-on-Chips: Microfluidic systems have been used to create "organs-on-chips," which are microscale models of human organs. These systems replicate the physiological conditions of specific organs, allowing researchers to study organ function, disease progression, and drug responses in a more realistic and controlled environment.
Drug Discovery and Development: Microfluidics has revolutionized drug discovery by providing a platform for high-throughput screening of compounds, studying drug interactions, and optimizing drug formulations. These devices enable more efficient and cost-effective drug development processes.
Single-Cell Analysis: Microfluidic devices have facilitated the analysis of individual cells, allowing researchers to study cellular heterogeneity and understand the behavior of individual cells in a population. This is crucial for advancements in fields like cancer research, immunology, and neuroscience.
Synthetic Biology Applications: Microfluidics plays a role in synthetic biology by providing precise control over the delivery of reagents, facilitating the construction of artificial biological systems. This is important for the development of bioengineered organisms and the synthesis of novel biomolecules.
Environmental Monitoring: Microfluidic devices have been used for on-site monitoring of environmental parameters, including water quality, air pollution, and soil analysis. These devices offer rapid and portable solutions for environmental monitoring in various settings.
Tissue Engineering and Regenerative Medicine: Microfluidics has contributed to the field of tissue engineering by providing platforms for creating and maintaining 3D cell cultures. This is essential for developing artificial tissues and organs for transplantation.
Tags
Smart Materials Conferences 2024 Aisa
Smart Materials Conferences 2024 Europe
Materials Science Conferences 2024 Europe
Intelligent Materials Conferences
Nanotechnology Conferences 2024 USA
Smart Materials Conferences 2024 USA
Nanotechnology Conferences 2024 Europe
Smart Materials Conferences 2024 Japan
Nanotechnology Conferences 2024
Materials Science Conferences
Materials Science Conferences 2024 Middle East
Artificial Materials Conferences
Nanotechnology Conferences 2024 Middle East
Smart Materials Conferences
Nanocomposites are materials composed of a matrix (polymer, metal, or ceramic) reinforced with nanoscale particles, often referred to as nanofillers or nanoparticles. These materials exhibit improved properties compared to traditional composites due to the unique characteristics of the nanoparticles. The field of nanocomposites has seen significant advancements in recent years, leading to a wide range of applications across various industries. Here are some key aspects of nanocomposites and their advancements:
Enhanced Mechanical Properties: The addition of nanofillers, such as carbon nanotubes, graphene, or nanoclays, can significantly enhance the mechanical properties of nanocomposites. These materials demonstrate increased strength, stiffness, and toughness, making them suitable for structural applications in aerospace, automotive, and construction industries.
Improved Thermal and Electrical Conductivity: Nanocomposites often exhibit enhanced thermal and electrical conductivity due to the high aspect ratio and unique properties of the nanofillers. This makes them suitable for applications in electronics, thermal management, and energy storage.
Barrier Properties: Nanocomposites can provide improved barrier properties against gases, liquids, and other environmental factors. This is especially valuable in packaging materials, where nanocomposites can extend the shelf life of products and enhance the barrier against moisture and oxygen.
Flame Retardancy: Nanocomposites have been developed with improved flame-retardant properties, making them suitable for use in materials that require enhanced fire resistance, such as in the construction and transportation industries.
Biomedical Applications: Nanocomposites are finding applications in the biomedical field, particularly in the development of biocompatible materials for drug delivery, tissue engineering, and medical implants. The controlled release of drugs from nanocomposite materials can improve therapeutic outcomes.
Self-Healing Materials: Advances in nanocomposite research have led to the development of self-healing materials, where the nanofillers contribute to the material's ability to repair damage automatically. This has implications for extending the lifespan of materials and reducing maintenance requirements.
Lightweight Materials: The use of lightweight nanofillers in composites helps in reducing the overall weight of materials, making them attractive for applications where weight is a critical factor, such as in automotive and aerospace industries.
Multifunctional Properties: Nanocomposites can be designed with multifunctional properties by incorporating various types of nanofillers. For example, a single material could possess mechanical strength, electrical conductivity, and thermal resistance simultaneously.
Green and Sustainable Nanocomposites: Research is ongoing to develop nanocomposites using environmentally friendly materials and processes. This includes the use of bio-based polymers and sustainable nanofillers, contributing to the development of eco-friendly materials.
Tags
Materials Science Conferences 2024
Nanotechnology Conferences 2024 Europe
Materials Science Conferences
Carbon Materials Conferences
Artificial Materials Conferences
Materials Science Conferences 2024 Asia
Materials Science and Engineering Conferences
Intelligent Materials Conferences
Materials Science Conferences 2024 USA
Piezoelectric Materials Conferences
Advanced Materials Conferences
Materials Science Conferences 2024 Middle East
Smart Materials Conferences 2024 Europe
Nanotechnology Conferences 2024
Nanofibers are fibers with diameters in the nanometer range, typically between 1 and 100 nanometers. These fibers can be produced from various materials, including polymers, carbon, ceramics, and proteins. The unique properties of nanofibers, such as their high surface area, flexibility, and strength, have led to advancements in a variety of fields. Here are some key aspects of nanofibers and their recent advancements:
Electrospinning Technique: The most common method for producing nanofibers is electrospinning. This technique involves using an electric field to draw a thin fiber from a liquid polymer solution or melt. Advances in electrospinning technology have improved the precision, scalability, and versatility of the process, allowing the production of nanofibers with controlled dimensions and tailored properties.
Biomedical Applications: Nanofibers are extensively used in biomedical applications. They are employed in tissue engineering for scaffolds that mimic the extracellular matrix, promoting cell adhesion and growth. Nanofibers are also used for drug delivery systems, wound healing, and as antimicrobial materials.
Protective Clothing and Filtration: Nanofibers have been utilized in the development of protective clothing and filtration materials. The high surface area and porosity of nanofibers make them effective in filtering out particles, bacteria, and viruses. Applications include air and water filtration, as well as protective masks and clothing.
Electronic Devices: Nanofibers have been incorporated into electronic devices and sensors. They can be used in flexible electronics, wearable sensors, and as components in energy storage devices. Nanofibers with conductive properties, such as those made from carbon nanotubes or graphene, are particularly useful in these applications.
Energy Storage and Conversion: Nanofibers play a role in energy storage and conversion technologies. They are used in the development of electrodes for batteries, supercapacitors, and fuel cells. The high surface area of nanofibers enhances the efficiency of energy storage devices.
Textiles and Smart Fabrics: Nanofibers are integrated into textiles to enhance their properties. They contribute to the development of smart fabrics with functionalities such as sensing, self-cleaning, and thermal regulation. Nanofibrous materials are also used to create lightweight and durable textiles.
Environmental Remediation: Nanofibers have been employed in environmental applications, including the removal of pollutants from air and water. Functionalized nanofibers can selectively capture contaminants, making them useful for environmental remediation.
Optical Devices: Nanofibers are used in the development of optical devices and sensors. They can be engineered to exhibit specific optical properties, making them suitable for applications such as sensors, lasers, and imaging devices.
Tags
Smart Materials Conferences 2024 USA
Smart Materials Conferences 2024 Aisa
Smart Materials Conferences 2024 Japan
Nanotechnology Conferences 2024 USA
Nanotechnology Conferences 2024
Materials Science Conferences
Materials Science Conferences 2024 Europe
Smart Materials Conferences
Artificial Materials Conferences
Composite Materials Conferences
Materials Science Conferences 2024
Materials Science Conferences 2024 Asia
Smart Materials Conferences 2024 Europe
Nanotechnology Conferences 2024 Europe
Photovoltaic Materials Conferences
Nanoparticle-based sensors are devices that utilize nanoparticles to detect and respond to specific substances or environmental conditions. These sensors have gained significant attention due to the unique properties of nanoparticles, such as their high surface area, enhanced reactivity, and tunable electronic, optical, and magnetic characteristics. The advancements in nanoparticle-based sensors hold great potential for numerous applications, offering improved sensitivity, selectivity, and versatility. Here are some key aspects of nanoparticle-based sensors and their advancements:
Improved Sensitivity and Selectivity: Nanoparticles provide a large surface area for interactions with target molecules, leading to enhanced sensitivity in sensor responses. Functionalizing nanoparticles allows for specific binding to target analytes, improving the selectivity of sensors.
Biomedical Applications: Nanoparticle-based sensors are extensively used in the biomedical field for diagnostic purposes. They play a crucial role in the detection of biomarkers, pathogens, and various disease-related molecules. This has implications for early disease diagnosis and monitoring.
Environmental Monitoring: Nanoparticle-based sensors contribute to environmental monitoring by detecting pollutants, toxins, and other environmental contaminants. These sensors can be deployed in air, water, and soil to provide real-time data for environmental quality assessment.
Food Safety and Quality Control: Nanoparticle-based sensors are employed in the food industry for the rapid and sensitive detection of contaminants, pathogens, and spoilage indicators. This helps ensure food safety and quality throughout the supply chain.
Explosive and Chemical Detection: Nanoparticle-based sensors are utilized for the detection of explosives and harmful chemicals. The high sensitivity of these sensors allows for the identification of trace amounts of substances, enhancing security measures in various settings.
Gas Sensing: Nanoparticles with unique electronic and catalytic properties are used in gas sensors for detecting specific gases. These sensors find applications in industrial safety, environmental monitoring, and medical diagnostics.
Point-of-Care Testing (POCT): Nanoparticle-based sensors are crucial for point-of-care testing, enabling rapid and on-site diagnostics. They are particularly valuable in resource-limited settings where access to traditional laboratory facilities is limited.
Remote Sensing and Imaging: Nanoparticle-based sensors are integrated into imaging technologies for remote sensing applications. These sensors enable the detection of specific targets in remote or inaccessible areas, supporting various fields, including environmental monitoring and defense.
Real-Time Monitoring and IoT Integration: Nanoparticle-based sensors contribute to the development of real-time monitoring systems and integrate seamlessly with the Internet of Things (IoT). This connectivity enhances data collection, analysis, and response capabilities.
Tags
Composite Materials Conferences
Materials Science and Engineering Conferences
Materials Science Conferences 2024 Asia
Photovoltaic Materials Conferences
Smart Materials Conferences 2024 Europe
Nanotechnology Conferences 2024
Artificial Materials Conferences
Nanotechnology Conferences 2024 Middle East
Materials Science Conferences 2024 Middle East
Materials Science Conferences 2024 USA
Carbon Materials Conferences
Piezoelectric Materials Conferences
Nanotechnology Conferences 2024 Asia
Nanotechnology Conferences 2024 Europe
Smart Materials Conferences 2024 USA
Nanostructured catalysts are catalytic materials with dimensions at the nanoscale. The design and engineering of catalysts at this level offer unique properties and advantages due to the increased surface area, enhanced reactivity, and tunable characteristics of nanomaterials. Breakthroughs in nanostructured catalysts have had a profound impact on various industries, particularly in the fields of energy production, environmental remediation, and chemical synthesis. Here are key aspects of nanostructured catalysts and some breakthroughs:
Increased Surface Area: One of the primary advantages of nanostructured catalysts is their significantly increased surface area per unit volume compared to bulk materials. This large surface area provides more active sites for catalytic reactions, leading to enhanced catalytic activity.
Improved Catalytic Efficiency: Nanostructured catalysts often exhibit higher catalytic efficiency and selectivity, enabling more effective conversion of reactants into desired products. This is crucial in industrial processes to optimize resource utilization and reduce waste.
Energy Conversion and Storage: Nanostructured catalysts play a crucial role in energy-related applications, such as fuel cells, batteries, and hydrogen production. Breakthroughs in these areas involve the development of nanomaterials that enhance reaction kinetics, increase energy density, and improve the overall efficiency of energy conversion and storage devices.
Environmental Applications: Nanostructured catalysts are employed in environmental remediation processes for the removal of pollutants from air and water. Breakthroughs in this area include the development of catalysts with high efficiency in degrading organic pollutants and converting harmful gases into less toxic substances.
Catalytic Nanomaterials for Renewable Energy: Advancements in nanostructured catalysts contribute to the development of sustainable and renewable energy technologies. These catalysts play a crucial role in processes like photocatalysis for water splitting, where sunlight is used to generate hydrogen as a clean energy carrier.
Selective Catalysis: Nanostructured catalysts allow for more precise control over catalytic reactions, leading to breakthroughs in selective catalysis. This is particularly important in the pharmaceutical and fine chemical industries, where precise control over reaction pathways is essential for synthesizing specific products.
Heterogeneous Catalysis: Nanostructured catalysts are often used in heterogeneous catalysis, where the catalyst and reactants are in different phases. Breakthroughs in this area involve the design of catalysts with improved stability, recyclability, and performance in various reaction conditions.
Single-Atom Catalysis: Breakthroughs in nanotechnology have enabled the development of single-atom catalysts, where individual metal atoms act as catalytic sites. This approach maximizes atom efficiency and can lead to increased catalytic activity and selectivity.
Tags
Smart Materials Conferences 2024 USA
Materials Science Conferences 2024 USA
Nanotechnology Conferences 2024 USA
Materials Science Conferences 2024 Middle East
Smart Materials Conferences
Intelligent Materials Conferences
Carbon Materials Conferences
Materials Science Conferences 2024 Europe
Nanotechnology Conferences 2024 Middle East
Composite Materials Conferences
Smart Materials Conferences 2024 Europe
Piezoelectric Materials Conferences
Photovoltaic Materials Conferences
Advanced Materials Conferences
Smart Materials Conferences 2024 Aisa
Photovoltaic materials are substances used in the construction of solar cells and panels to convert sunlight into electrical energy. These materials play a crucial role in the efficiency and performance of solar photovoltaic devices. Advances in photovoltaic materials have been central to improving the efficiency, cost-effectiveness, and overall viability of solar energy as a sustainable power source. Here are key aspects of photovoltaic materials and their advancements:
Crystalline Silicon (c-Si) Solar Cells: Traditional solar cells predominantly use crystalline silicon as the active material. Advances in c-Si technology include improvements in crystal growth techniques, such as the development of monocrystalline and multicrystalline silicon, as well as the enhancement of manufacturing processes to reduce costs and increase efficiency.
Thin-Film Solar Cells: Thin-film solar cells utilize materials like amorphous silicon (a-Si), cadmium telluride (CdTe), and copper indium gallium selenide (CIGS). Advances in thin-film technology involve the optimization of deposition techniques, the development of flexible and lightweight substrates, and improvements in the stability and efficiency of thin-film solar modules.
Perovskite Solar Cells: Perovskite solar cells have gained attention for their rapid efficiency improvements and low-cost fabrication. Advances in perovskite technology include increased stability, scalability, and the exploration of tandem cell configurations (combining perovskite with other materials) to achieve even higher efficiencies.
Organic Photovoltaic Materials: Organic photovoltaic (OPV) materials involve the use of organic compounds, such as polymers or small molecules, as the active material in solar cells. Advancements in OPV materials focus on improving the power conversion efficiency, stability, and scalability of organic solar cells for applications like flexible and lightweight solar panels.
Tandem Solar Cells: Tandem solar cells combine multiple layers of different photovoltaic materials to capture a broader range of sunlight and increase overall efficiency. Advances in tandem solar cells involve the optimization of material combinations, interfaces, and fabrication processes to achieve higher efficiencies than single-junction cells.
Quantum Dot Solar Cells: Quantum dots, semiconductor nanocrystals, have unique optical and electronic properties that make them suitable for solar cells. Advances in quantum dot solar cells include the development of efficient quantum dot sensitizers for use in next-generation photovoltaic devices.
Transparent Conductive Films: Transparent conductive films, often based on materials like indium tin oxide (ITO), are crucial for allowing light to reach the active material while facilitating electron transport. Advances in transparent conductive films focus on finding alternatives to indium-based materials, such as flexible and transparent conductive polymers or metal mesh structures.
Advanced Antireflection Coatings: Antireflection coatings are essential to minimize light reflection and maximize light absorption in solar cells. Advances in antireflection coatings involve the development of novel materials and coatings that enhance light trapping and improve overall device performance.
Tandem Perovskite-Silicon Solar Cells: Combining perovskite and crystalline silicon in tandem solar cells is a promising approach to achieve high efficiencies. Advances in this area focus on overcoming stability challenges, optimizing the tandem structure, and addressing material compatibility issues between perovskite and silicon.
Tags
Smart Materials Conferences 2024
Materials Science Conferences 2024 Europe
Nanotechnology Conferences 2024
Piezoelectric Materials Conferences
Composite Materials Conferences
Smart Materials Conferences 2024 USA
Smart Materials Conferences 2024 Aisa
Materials Science Conferences
Smart Materials Conferences 2024 Japan
Nanotechnology Conferences 2024 Asia
Nanotechnology Conferences 2024 Europe
Nanotechnology Conferences 2024 Middle East
Artificial Materials Conferences
Materials Science Conferences 2024
Smart Materials Conferences
pH-responsive polymers, also known as smart or stimuli-responsive polymers, are a class of materials that undergo changes in their physical or chemical properties in response to variations in pH (acidity or alkalinity). These polymers can be designed to swell, shrink, or undergo conformational changes based on the pH of their environment. The advancements in pH-responsive polymers have led to the development of materials with diverse applications in drug delivery, sensors, coatings, and other fields. Here are key aspects of pH-responsive polymers and their advancements:
Drug Delivery Systems: pH-responsive polymers are widely utilized in drug delivery applications. These polymers can respond to the pH gradients in different body tissues or cellular compartments, releasing drugs in a controlled and targeted manner. Advances in this area include the design of polymers that respond to specific pH conditions associated with diseased tissues, such as tumors or inflamed regions.
Gastrointestinal Drug Delivery: pH-responsive polymers are employed for drug delivery in the gastrointestinal tract, where pH levels vary. Polymers can be designed to release drugs in the stomach or the intestine based on the pH conditions of these regions, improving drug absorption and bioavailability.
Hydrogels for Wound Healing: pH-responsive hydrogels are developed for wound healing applications. These hydrogels can change their swelling behavior based on the pH of the wound environment, promoting optimal conditions for tissue regeneration. pH-responsive hydrogels are also used in contact lenses for enhanced comfort.
Responsive Nanoparticles: Advances in nanotechnology have led to the development of pH-responsive nanoparticles. These nanoparticles can encapsulate drugs or imaging agents and release them in response to changes in pH, making them suitable for targeted drug delivery and imaging applications.
Food Packaging Materials: pH-responsive polymers are explored for use in intelligent food packaging materials. These materials can change permeability or indicator color based on the pH of the food, providing information about food freshness and spoilage.
Advancements in Polymer Design: Researchers are continuously exploring new polymer architectures and compositions to enhance the responsiveness of pH-sensitive polymers. This includes the development of multi-stimuli-responsive polymers that can respond to multiple environmental cues.
Microfluidics and Lab-on-a-Chip Technologies: pH-responsive polymers are integrated into microfluidic devices and lab-on-a-chip technologies for applications in chemical analysis, diagnostics, and controlled reactions. These devices can exploit the pH responsiveness of polymers for precise control over fluid flow and reactions.
Tags
Piezoelectric Materials Conferences
Smart Materials Conferences 2024
Smart Materials Conferences 2024 Europe
Carbon Materials Conferences
Advanced Materials Conferences
Smart Materials Conferences 2024 Japan
Nanotechnology Conferences 2024 Middle East
Nanotechnology Conferences 2024
Intelligent Materials Conferences
Nanotechnology Conferences 2024 Asia
Nanotechnology Conferences 2024 Europe
Composite Materials Conferences
Materials Science Conferences 2024 Europe
Nanotechnology Conferences 2024 USA
Materials Science Conferences 2024 USA
Piezoelectric materials are a type of material that can generate an electric charge in response to mechanical stress, and vice versa—they can deform or change shape when subjected to an electric field. This phenomenon is known as the piezoelectric effect. These materials have unique properties that make them useful in various applications, ranging from sensors and actuators to energy harvesting devices.
Breakthroughs in piezoelectric materials have occurred over the years, leading to advancements in technology and the development of new applications. Some notable breakthroughs include:
Lead Zirconate Titanate (PZT): PZT is a commonly used piezoelectric material due to its high piezoelectric coefficients and versatility. Breakthroughs in understanding the crystal structures and compositions of PZT have improved its performance in applications like sensors and actuators.
Flexible and Organic Piezoelectric Materials: Researchers have made strides in developing flexible and organic piezoelectric materials, expanding their use in wearable devices, biomedical applications, and energy harvesting from ambient vibrations. These materials offer flexibility and lightweight characteristics not found in traditional piezoelectrics.
Two-Dimensional (2D) Materials: Some 2D materials, such as graphene and transition metal dichalcogenides (TMDs), have exhibited piezoelectric properties. This breakthrough opens up possibilities for incorporating piezoelectricity into extremely thin and lightweight materials, potentially revolutionizing the design of electronic devices.
Lead-Free Piezoelectric Materials: Due to environmental concerns, there has been significant research to find lead-free alternatives to traditional piezoelectric materials. Breakthroughs in developing lead-free materials with comparable or superior piezoelectric properties have been achieved, addressing environmental and health issues associated with lead-containing materials.
Biomedical Applications: Advances in piezoelectric materials have led to innovative biomedical applications, including ultrasound imaging, drug delivery systems, and even energy harvesting within the human body for medical implants.
Research in piezoelectric materials continues, with ongoing efforts to enhance their performance, explore new compositions, and discover novel applications across various industries. These breakthroughs contribute to the expanding role of piezoelectric materials in modern technology.
Tags
Materials Science and Engineering Conferences
Composite Materials Conferences
Nanotechnology Conferences 2024 Middle East
Photovoltaic Materials Conferences
Nanotechnology Conferences 2024 USA
Materials Science Conferences 2024 USA
Smart Materials Conferences 2024 Japan
Materials Science Conferences 2024 Asia
Advanced Materials Conferences
Piezoelectric Materials Conferences
Smart Materials Conferences 2024 Europe
Materials Science Conferences 2024
Materials Science Conferences 2024 Europe
Smart Materials Conferences 2024 Aisa
Materials Science Conferences
Quantum dots are semiconductor nanoparticles that exhibit quantum mechanical properties. These nanoscale structures have unique optical and electronic characteristics, making them valuable in a variety of applications, including displays, solar cells, biological imaging, and quantum computing. Quantum dots are typically composed of semiconductor materials like cadmium selenide (CdSe) or indium arsenide (InAs), and their size dictates their electronic and optical properties.
Advancements in quantum dot technology have been substantial, leading to improved performance and expanded applications. Some notable advancements include:
Improved Quantum Yield: Quantum yield refers to the efficiency with which quantum dots emit light. Researchers have made significant strides in enhancing the quantum yield of quantum dots, resulting in brighter and more efficient light emission. This improvement is crucial for applications such as displays and lighting.
Tunable Emission Wavelengths: One of the key advantages of quantum dots is their tunable emission wavelengths. Researchers have developed methods to precisely control the size of quantum dots during synthesis, allowing for fine-tuning of the emitted light's color. This tunability is essential for applications in displays and biological imaging.
Cd-Free and Non-Toxic Quantum Dots: To address environmental and health concerns associated with cadmium-containing quantum dots, researchers have worked on developing Cd-free alternatives. Non-toxic quantum dots, often made of materials like indium phosphide (InP) or silicon, have been successfully synthesized and are gaining attention for various applications.
Biomedical Imaging and Drug Delivery: Quantum dots have found applications in biological imaging and drug delivery due to their unique optical properties. Advances in surface modification and biofunctionalization techniques have enabled better targeting, biocompatibility, and imaging capabilities, making them valuable tools in medical research and diagnostics.
Quantum Dot Solar Cells: Quantum dots have been incorporated into solar cells to enhance their efficiency. Researchers have explored the use of quantum dots in photovoltaic devices to capture a broader range of solar wavelengths and improve the overall conversion of sunlight into electricity.
Quantum Dot Displays: Quantum dots are used in display technologies, such as Quantum Dot Light Emitting Diodes (QLEDs) and Quantum Dot Enhancement Films (QDEF). These displays offer improved color accuracy, wider color gamuts, and better energy efficiency compared to traditional display technologies.
Quantum Dot Cellular Automata (QCA): In the realm of quantum computing, quantum dots have been investigated for their potential use in QCA, a paradigm for building quantum computers. Quantum dots can serve as qubits, the basic units of quantum information, in certain quantum computing architectures.
Tags
Materials Science Conferences 2024 Europe
Nanotechnology Conferences 2024 Middle East
Carbon Materials Conferences
Nanotechnology Conferences 2024 Asia
Advanced Materials Conferences
Smart Materials Conferences 2024 Aisa
Piezoelectric Materials Conferences
Artificial Materials Conferences
Materials Science Conferences 2024 USA
Nanotechnology Conferences 2024
Materials Science Conferences 2024 Middle East
Smart Materials Conferences 2024 USA
Composite Materials Conferences
Intelligent Materials Conferences
Photovoltaic Materials Conferences
Responsive drug delivery systems refer to drug delivery platforms that can modulate the release of therapeutic agents in response to specific stimuli. These stimuli can be internal or external triggers, such as changes in pH, temperature, light, or the presence of specific biomolecules. The goal of responsive drug delivery is to enhance the precision and control of drug release, improving therapeutic efficacy while minimizing side effects. Breakthroughs in this field have led to innovative approaches in personalized medicine and targeted drug delivery. Some key aspects and breakthroughs include:
pH-Responsive Systems: pH-responsive drug delivery systems take advantage of variations in acidity within the body. For example, the slightly acidic environment of tumors can be targeted by pH-sensitive nanocarriers. Breakthroughs include the development of smart polymers and nanoparticles that release drugs specifically in response to the pH of the tumor microenvironment, improving drug delivery to cancer cells while sparing healthy tissues.
Temperature-Responsive Systems: Temperature-sensitive drug delivery systems respond to changes in temperature. These systems can be designed to release drugs when exposed to specific temperature ranges, such as the elevated temperatures associated with inflammation or infection. Breakthroughs in this area involve the design of thermosensitive polymers and liposomes for controlled drug release.
Light-Responsive Systems: Light-triggered drug delivery relies on photosensitive materials that release drugs upon exposure to light of a specific wavelength. This approach allows for precise spatiotemporal control over drug release. Advances include the use of light-responsive nanoparticles and photoresponsive molecules for targeted drug delivery in various tissues.
Enzyme-Responsive Systems: Enzyme-responsive drug delivery systems exploit the presence of specific enzymes in pathological conditions. For instance, certain diseases exhibit elevated levels of particular enzymes. Breakthroughs involve the design of drug carriers that are sensitive to these enzymes, enabling triggered drug release at the disease site.
Magnetic-Responsive Systems: Magnetic-responsive drug delivery systems use magnetic fields to trigger drug release. Magnetic nanoparticles can be incorporated into drug carriers, allowing for remotely controlled drug delivery. This approach has applications in cancer therapy, where magnetic fields can guide drug-loaded nanoparticles to the tumor site.
Bioresponsive Hydrogels: Hydrogels that respond to physiological conditions or specific biomolecules have gained attention in drug delivery. These hydrogels can swell or contract in response to changes in temperature, pH, or the presence of biomarkers, facilitating controlled drug release. Breakthroughs involve the development of bioresponsive hydrogels for applications in wound healing, tissue engineering, and drug delivery.
Personalized Medicine: The integration of responsive drug delivery systems with advances in genomics and diagnostics has paved the way for personalized medicine. Tailoring drug delivery to individual patient characteristics and disease profiles can optimize therapeutic outcomes and minimize adverse effects.
Tags
Smart Materials Conferences 2024 Aisa
Materials Science Conferences
Smart Materials Conferences 2024 Europe
Artificial Materials Conferences
Nanotechnology Conferences 2024 Middle East
Advanced Materials Conferences
Materials Science and Engineering Conferences
Smart Materials Conferences
Piezoelectric Materials Conferences
Composite Materials Conferences
Materials Science Conferences 2024 USA
Intelligent Materials Conferences
Smart Materials Conferences 2024 Japan
Nanotechnology Conferences 2024 Europe
Smart Materials Conferences 2024 USA
Responsive membranes are materials that can dynamically change their properties or behavior in response to external stimuli. These stimuli can include changes in temperature, pH, pressure, light, or the presence of specific ions or molecules. The ability of membranes to respond to such stimuli is harnessed for various applications, including water purification, drug delivery, sensing, and controlled release systems. Advancements in responsive membranes have led to improvements in performance, selectivity, and adaptability in diverse fields. Here are some key aspects and breakthroughs in responsive membranes:
Smart Polymer Membranes: Responsive membranes often utilize smart polymers, which undergo reversible changes in their structure or properties in response to external stimuli. For example, stimuli-responsive hydrogels can change their swelling behavior based on changes in pH or temperature. These polymers are incorporated into membranes to achieve responsive characteristics.
Temperature-Responsive Membranes: Membranes that respond to changes in temperature have applications in controlled drug delivery and separation processes. Advances include the development of temperature-responsive polymers that can undergo phase transitions, altering the membrane's permeability in response to temperature changes.
Ion-Responsive Membranes: Membranes responsive to specific ions play a role in applications like ion-selective sensors and controlled ion transport. Advanced ion-responsive membranes have been developed to selectively transport ions based on changes in the external ion concentration or composition.
Light-Responsive Membranes: Light-responsive membranes can change their properties or allow controlled release in response to exposure to light. This is particularly useful in drug delivery and controlled release systems where spatiotemporal control is desired. Breakthroughs involve the incorporation of photoresponsive materials into membrane structures.
Molecularly Imprinted Membranes: Molecularly imprinted membranes are designed to selectively recognize and bind specific molecules. The imprinted sites on the membrane can be responsive to changes in the target molecule's concentration, enabling applications in sensing and separation.
Self-Healing Membranes: Breakthroughs in materials science have led to the development of self-healing membranes that can repair damage or defects autonomously. This is particularly beneficial in applications where membrane integrity is critical, such as water purification and gas separation.
Adaptive Membranes for Water Treatment: Responsive membranes are employed in water treatment processes to enhance selectivity and efficiency. These membranes can adapt to changes in water quality and fouling conditions, leading to improved performance and longer operational lifetimes.
Electroresponsive Membranes: Membranes responsive to electrical stimuli have applications in fields such as drug delivery and electrochemical sensing. These membranes can change their permeability or structure in response to applied electrical fields.
Tags
Nanotechnology Conferences 2024 Middle East
Materials Science Conferences 2024 Asia
Nanotechnology Conferences 2024
Nanotechnology Conferences 2024 USA
Advanced Materials Conferences
Piezoelectric Materials Conferences
Smart Materials Conferences 2024 Japan
Materials Science Conferences 2024 Middle East
Artificial Materials Conferences
Smart Materials Conferences
Materials Science Conferences 2024
Photovoltaic Materials Conferences
Intelligent Materials Conferences
Smart Materials Conferences 2024 Aisa
Materials Science Conferences 2024 Europe
Responsive surfaces for anti-fouling applications involve the development of materials that can dynamically adapt their properties to resist the adhesion of unwanted substances, such as biofouling, microbial growth, or the accumulation of contaminants. The goal is to create surfaces that actively prevent fouling by responding to environmental cues or external stimuli. Breakthroughs in this field have led to advancements in marine coatings, medical devices, and various industrial applications. Here are some key aspects and breakthroughs in responsive surfaces for anti-fouling applications:
Bio-Inspired Designs: Breakthroughs in anti-fouling surfaces have been inspired by nature. For instance, researchers have studied the surfaces of marine organisms like sharks and mussels, which exhibit natural anti-fouling properties. Mimicking these biological surfaces has led to the development of coatings that can resist attachment by microorganisms and other fouling agents.
Superhydrophobic Surfaces: Superhydrophobic surfaces, which repel water, have been explored for anti-fouling applications. These surfaces prevent the accumulation of waterborne contaminants, and their self-cleaning properties can help reduce biofouling. Advances in fabrication techniques have led to durable and scalable superhydrophobic coatings.
Zwitterionic Materials: Zwitterionic materials have been used in the development of anti-fouling surfaces. These materials have both positive and negative charges, creating a balanced charge distribution that resists the adhesion of proteins and other fouling agents. Zwitterionic coatings are known for their effectiveness in preventing biofouling on medical devices and implants.
Responsive Polymers: Polymer-based materials that respond to changes in environmental conditions, such as pH or temperature, have been employed for anti-fouling purposes. Responsive polymers can undergo conformational changes that affect their surface properties, making them less prone to fouling.
Dynamic Release Systems: Breakthroughs in responsive surfaces include the development of dynamic release systems. These systems can release anti-fouling agents or coatings in response to specific stimuli, providing a continuous protection against fouling. This approach is particularly useful in marine applications.
Robust and Scalable Manufacturing Techniques: Advances in manufacturing techniques, such as scalable and cost-effective methods for applying responsive coatings, have contributed to the practical implementation of anti-fouling surfaces in real-world applications.
Environmental Sustainability: Researchers are increasingly focused on developing environmentally sustainable anti-fouling solutions that minimize the use of toxic substances. This includes the exploration of bio-based and eco-friendly materials for anti-fouling applications.
Tags
Smart Materials Conferences 2024 USA
Nanotechnology Conferences 2024 Europe
Piezoelectric Materials Conferences
Nanotechnology Conferences 2024
Carbon Materials Conferences
Composite Materials Conferences
Nanotechnology Conferences 2024 USA
Smart Materials Conferences 2024 Europe
Materials Science Conferences
Nanotechnology Conferences 2024 Asia
Nanotechnology Conferences 2024 Middle East
Artificial Materials Conferences
Materials Science Conferences 2024 USA
Smart Materials Conferences
Materials Science Conferences 2024
Self-cleaning surfaces refer to materials or coatings that have the ability to repel or remove dirt, contaminants, and other unwanted substances without the need for external cleaning. These surfaces often leverage various physical or chemical mechanisms to achieve self-cleaning properties. Advancements in self-cleaning surfaces have been driven by the desire for low-maintenance, environmentally friendly materials in applications ranging from architecture to consumer products. Here are some key aspects and breakthroughs in the field:
Hydrophobic and Superhydrophobic Surfaces: Hydrophobic surfaces repel water, while superhydrophobic surfaces exhibit extreme water repellency and self-cleaning properties. Advances in fabricating superhydrophobic coatings have led to the development of materials that can repel water and prevent the adhesion of dirt and contaminants. These surfaces are used in applications such as building materials, textiles, and electronics.
Photocatalytic Coatings: Photocatalysis involves using light to trigger chemical reactions. Photocatalytic coatings, often based on materials like titanium dioxide (TiO2), harness sunlight to break down organic pollutants on the surface. This self-cleaning mechanism has been employed in architectural coatings and outdoor surfaces.
Lotus Effect: Inspired by the lotus leaf, which remains clean due to its micro-nanostructured surface and water-repellent wax, the Lotus Effect has been mimicked in self-cleaning materials. Advances in surface engineering have allowed for the creation of lotus-inspired coatings that resist dirt and water.
Oleophobic Surfaces: Oleophobic surfaces repel oils and greases, preventing their adhesion. Advancements in oleophobic coatings have applications in various industries, including consumer electronics where fingerprint-resistant surfaces are desired.
Nanotechnology in Self-Cleaning Materials: Nanotechnology plays a crucial role in the development of self-cleaning surfaces. Nanoscale structures and coatings enable enhanced water repellency, reduced surface energy, and improved resistance to contaminants. Nanomaterials like nanoparticles and nanotextures contribute to the effectiveness of self-cleaning materials.
Durable and Long-Lasting Coatings: Breakthroughs in the durability of self-cleaning coatings have been achieved through improved materials and manufacturing techniques. Long-lasting coatings are essential for practical applications, especially in outdoor environments where exposure to weathering and abrasion is significant.
Bionic and Bio-Inspired Designs: Researchers have drawn inspiration from natural self-cleaning mechanisms observed in animals and plants. Bio-inspired designs, such as those mimicking the structure of butterfly wings or gecko feet, have led to the development of self-cleaning surfaces with unique properties.
Application in Solar Panels: Self-cleaning coatings on solar panels can prevent the accumulation of dust and dirt, improving energy efficiency by maintaining the transparency of the panel surfaces. This application is crucial in regions with arid climates.
Antimicrobial Self-Cleaning Surfaces: Combining self-cleaning properties with antimicrobial features, certain surfaces can resist microbial growth, making them suitable for healthcare settings, food processing, and public spaces.
Tags
Nanotechnology Conferences 2024 USA
Materials Science and Engineering Conferences
Intelligent Materials Conferences
Artificial Materials Conferences
Materials Science Conferences 2024 Europe
Smart Materials Conferences 2024 Europe
Materials Science Conferences 2024 Asia
Smart Materials Conferences 2024 USA
Materials Science Conferences 2024
Advanced Materials Conferences
Materials Science Conferences 2024 Middle East
Nanotechnology Conferences 2024 Europe
Smart Materials Conferences
Photovoltaic Materials Conferences
Nanotechnology Conferences 2024
Self-healing polymers are materials capable of autonomously repairing damage or cracks without the need for external intervention. These polymers possess the ability to reestablish their structural integrity and functionality after experiencing mechanical damage, such as cuts, scratches, or fractures. Advancements in self-healing polymers have the potential to revolutionize various industries by extending the lifespan and durability of materials, reducing maintenance costs, and enhancing safety. Here are some key aspects and advancements in the field of self-healing polymers:
Microcapsule-Based Systems: One approach to self-healing polymers involves embedding microcapsules containing a healing agent within the polymer matrix. When the material is damaged, the microcapsules rupture, releasing the healing agent, which then fills the crack or void and undergoes polymerization to restore the material's integrity. Advances in microencapsulation techniques have improved the efficiency and reliability of self-healing systems.
Chemical Bond Exchange: Self-healing polymers can also rely on reversible chemical reactions to mend damage. For instance, dynamic covalent bonds, such as disulfide bonds or Diels-Alder reactions, can break and reform in response to mechanical stress, allowing the polymer to self-heal. Advances in designing polymers with dynamic bonds have led to materials with enhanced healing capabilities and mechanical properties.
Intrinsic Healing Mechanisms: Some polymers possess intrinsic self-healing capabilities without the need for external additives or triggers. These polymers can undergo molecular rearrangements or physical processes, such as chain diffusion or hydrogen bonding, to heal damage. Advancements in understanding the molecular mechanisms of intrinsic healing have facilitated the development of novel self-healing materials.
Autonomous Healing: Autonomous healing refers to the ability of polymers to heal damage without external stimuli or triggers. Self-healing polymers with autonomous healing properties can continuously monitor their structural integrity and initiate healing processes as needed, enhancing their reliability and applicability in real-world conditions.
Responsive and Triggered Healing: Self-healing polymers can be engineered to respond to specific stimuli, such as changes in temperature, pH, or light, to initiate healing. Triggered healing systems allow for precise control over the healing process, enabling on-demand repair of damaged materials. Advances in responsive polymers and stimuli-responsive triggers have expanded the capabilities of self-healing materials.
Electronics and Flexible Devices: Self-healing polymers are utilized in flexible electronics and wearable devices to prevent damage from bending, stretching, or impact. These materials enable the development of robust and long-lasting electronic components for diverse applications.
Future Directions: Ongoing research in self-healing polymers focuses on improving healing efficiency, scalability, and compatibility with existing manufacturing processes. By addressing challenges such as healing time, mechanical properties, and cost-effectiveness, advancements in self-healing materials are poised to have a profound impact on various industries and contribute to the advancement of materials science.
Tags
Smart Materials Conferences 2024 Japan
Intelligent Materials Conferences
Materials Science Conferences
Carbon Materials Conferences
Materials Science Conferences 2024 Middle East
Composite Materials Conferences
Materials Science Conferences 2024
Smart Materials Conferences 2024 USA
Photovoltaic Materials Conferences
Piezoelectric Materials Conferences
Materials Science Conferences 2024 Europe
Materials Science Conferences 2024 USA
Materials Science and Engineering Conferences
Smart Materials Conferences 2024 Europe
Nanotechnology Conferences 2024
Shape Memory Alloys (SMAs) are a class of metallic materials that can "remember" and recover their original shape or form after being deformed. This unique property is attributed to a solid-state phase transformation that occurs in the material. The most commonly used shape memory alloys are based on nickel-titanium (NiTi) compositions, but other alloys such as copper-aluminum-nickel and iron-manganese-silicon also exhibit shape memory effects.
Breakthroughs in the development and understanding of shape memory alloys have led to diverse applications across various industries. Here are key aspects and breakthroughs related to shape memory alloys:
Shape Memory Effect (SME): The shape memory effect is the defining characteristic of shape memory alloys. It involves the ability of the material to undergo reversible deformation at certain temperatures. When deformed at a low temperature, the alloy retains the deformed shape. Upon heating, it reverts to its original shape.
Superelasticity: In addition to the shape memory effect, many shape memory alloys exhibit superelasticity, also known as the pseudoelasticity. This property allows the material to undergo large deformations and then recover its original shape upon unloading, even without a phase transformation.
Applications in Biomedicine: One of the significant breakthroughs in shape memory alloys is their widespread use in biomedical applications. NiTi alloys are biocompatible and corrosion-resistant, making them suitable for medical implants such as stents, orthopedic devices, and guidewires. The ability to design devices that can be delivered in a compact form and then deployed in the body is a major advancement.
Actuators and Robotics: Shape memory alloys find applications in actuators and robotics due to their ability to produce large deformations with minimal input energy. They are used in various robotic systems, including grippers, valves, and morphing structures for adaptive and shape-changing devices.
Smart Materials in Aerospace: SMAs have been integrated into aerospace components for applications such as variable geometry wings, adaptive airfoil surfaces, and deployable structures. These materials contribute to improved aerodynamics, fuel efficiency, and overall performance of aerospace systems.
Damping and Vibration Control: Shape memory alloys have been employed for their damping properties in structural elements to control vibrations and reduce noise. This is particularly valuable in applications where traditional damping materials may be impractical.
Textile Industry: SMAs have found applications in the textile industry for the development of smart fabrics and responsive clothing. Shape memory alloys can be integrated into textiles to create self-adjusting garments, such as self-tightening shoe laces or shape-changing apparel.
Seismic Dampers: In civil engineering, shape memory alloys are used as seismic dampers to mitigate the impact of earthquakes on structures. These dampers absorb and dissipate energy during seismic events, reducing structural damage.
Miniaturization and Microactuators: Advances in manufacturing techniques and materials design have enabled the miniaturization of shape memory alloy-based devices. This is particularly important for microactuators and devices in fields like microelectronics and microsystems.
Improved Processing Techniques: Breakthroughs in the processing of shape memory alloys, including heat treatment methods and alloy compositions, have led to materials with enhanced properties, such as improved fatigue resistance and transformation temperatures tailored for specific applications.
Research on New Alloy Compositions: Ongoing research explores new alloy compositions and combinations to improve the performance and versatility of shape memory alloys. Researchers are investigating alternative elements and processing methods to tailor the properties of these materials for specific applications.
Tags
Smart Materials Conferences 2024 Aisa
Materials Science Conferences 2024 Europe
Materials Science and Engineering Conferences
Materials Science Conferences 2024 USA
Nanotechnology Conferences 2024 USA
Smart Materials Conferences 2024 USA
Materials Science Conferences 2024
Smart Materials Conferences
Artificial Materials Conferences
Nanotechnology Conferences 2024
Composite Materials Conferences
Nanotechnology Conferences 2024 Middle East
Smart Materials Conferences 2024 Europe
Nanotechnology Conferences 2024 Asia
Photovoltaic Materials Conferences
Shape-changing materials, also known as morphing materials or smart materials, are substances that can alter their physical shape, structure, or properties in response to external stimuli such as temperature, light, electric fields, or magnetic fields. These materials have applications in various fields, including aerospace, robotics, healthcare, and consumer electronics. Advancements in shape-changing materials have led to innovative technologies and devices with improved adaptability and functionality. Here are key aspects and breakthroughs in the field:
Shape Memory Alloys (SMAs): SMAs are a type of shape-changing material that can "remember" and return to a predetermined shape after being deformed. Typically, these alloys undergo a reversible phase transformation in response to changes in temperature. Nitinol (Nickel Titanium Naval Ordnance Laboratory) is a well-known SMA that has found applications in medical devices (stents), actuators, and robotics.
Electroactive Polymers (EAPs): EAPs are materials that deform in response to an electric field. They are lightweight and can be used in applications such as artificial muscles, soft robotics, and haptic interfaces. Advances in the development of EAPs with improved actuation capabilities and response times contribute to the field of flexible and adaptable structures.
Liquid Crystal Elastomers (LCEs): LCEs are materials that combine the properties of liquid crystals and elastomers, allowing them to undergo reversible shape changes in response to external stimuli such as temperature or light. LCEs are used in applications such as soft robotics and adaptive optics. Breakthroughs include the development of LCEs with tunable mechanical properties and precise control over shape-changing behavior.
Magnetostrictive Materials: Magnetostrictive materials change shape in response to an applied magnetic field. These materials find applications in sensors, actuators, and vibration damping systems. Advances in magnetostrictive materials include the development of materials with improved magnetostrictive coefficients for more efficient actuation.
Thermoresponsive Polymers: Thermoresponsive polymers undergo reversible changes in their physical properties, such as volume or shape, in response to changes in temperature. These polymers have applications in controlled drug delivery, tissue engineering, and responsive textiles. Breakthroughs involve the design of polymers with precise temperature-triggered transitions and tailored responses.
Photoresponsive Materials: Photoresponsive materials change their properties or shape when exposed to light. Photoresponsive polymers and molecules are used in applications such as light-driven actuators and photonic devices. Advances include the development of materials with enhanced photoresponsiveness and control over shape-changing behavior.
Origami and Kirigami Structures: Origami and kirigami techniques, inspired by traditional paper-folding arts, are applied to create complex three-dimensional structures that can change shape. These techniques are explored in fields like robotics, where foldable and reconfigurable structures are desirable.
3D Printing of Shape-Changing Structures: Additive manufacturing, particularly 3D printing, has enabled the fabrication of intricate shape-changing structures with high precision. This advancement allows for the customization of complex geometries and the integration of multiple materials with different properties.
Tags
Smart Materials Conferences 2024 USA
Artificial Materials Conferences
Materials Science Conferences
Materials Science Conferences 2024
Nanotechnology Conferences 2024 Europe
Materials Science Conferences 2024 Europe
Smart Materials Conferences 2024 Europe
Photovoltaic Materials Conferences
Advanced Materials Conferences
Intelligent Materials Conferences
Carbon Materials Conferences
Smart Materials Conferences
Composite Materials Conferences
Nanotechnology Conferences 2024 Middle East
Smart Materials Conferences 2024 Japan
Smart coatings refer to advanced materials that can respond to external stimuli by exhibiting specific functionalities or properties. These coatings are designed to be responsive to changes in the environment, such as temperature, light, pH, or the presence of certain substances. Breakthroughs in smart coatings have led to innovations in various industries, offering improved performance, durability, and functionality. Here are key aspects and breakthroughs in the field of smart coatings:
Self-Healing Coatings: Self-healing coatings have the ability to repair damage autonomously, extending the lifespan of surfaces and reducing maintenance costs. Microcapsules containing healing agents or polymers with reversible chemical bonds are incorporated into the coating, allowing it to recover from scratches, cracks, or other forms of damage.
Anti-Corrosion Coatings: Smart anti-corrosion coatings are designed to provide corrosion protection for metals. These coatings can detect early signs of corrosion and release inhibitors or healing agents to prevent further damage. This breakthrough is crucial for enhancing the durability of infrastructure, pipelines, and metal components.
Temperature-Responsive Coatings: Coatings that respond to changes in temperature can alter their properties, such as color or surface texture, depending on the environmental conditions. These coatings find applications in various fields, including smart windows that can modulate heat and light transmission based on temperature.
Photocatalytic Coatings: Photocatalytic coatings leverage light-induced chemical reactions to break down pollutants and contaminants on surfaces. This technology is applied in self-cleaning surfaces, air purification systems, and anti-microbial coatings.
Superhydrophobic and Oleophobic Coatings: Superhydrophobic coatings repel water, while oleophobic coatings repel oils and greases. These coatings have applications in self-cleaning surfaces, stain-resistant materials, and protective coatings for electronic devices.
Color-Changing Coatings: Color-changing coatings can adjust their color in response to external stimuli such as temperature, light, or pH. These coatings are used in applications like smart textiles, camouflage materials, and colorimetric sensors.
Responsive Antifouling Coatings: Antifouling coatings prevent the adhesion of marine organisms, bacteria, or contaminants to surfaces. Smart antifouling coatings can release bioactive substances or change their surface properties in response to environmental cues, enhancing their effectiveness.
pH-Responsive Coatings: Coatings that respond to changes in pH can find applications in controlled drug release systems, sensors, and biomedical devices. pH-responsive coatings can modulate their properties based on the acidity or alkalinity of their surroundings.
Electrochromic Coatings: Electrochromic materials change their color or optical properties in response to an applied electric field. Electrochromic coatings are used in smart windows, displays, and mirrors to control light transmission or reflectivity.
Antimicrobial Coatings: Smart coatings with antimicrobial properties can actively inhibit the growth of bacteria, fungi, or viruses on surfaces. These coatings are applied in healthcare settings, public spaces, and consumer products.
Mechanochromic Coatings: Mechanochromic coatings change color in response to mechanical deformation or stress. These coatings are used in damage detection, structural health monitoring, and as indicators for impact or strain.
Flexible and Stretchable Coatings: Breakthroughs in the development of coatings that can flex or stretch with the underlying substrate enable their use in flexible electronics, wearable devices, and other applications where traditional rigid coatings would not be suitable.
Tags
Materials Science Conferences 2024 USA
Materials Science Conferences 2024 Middle East
Nanotechnology Conferences 2024 Middle East
Intelligent Materials Conferences
Materials Science Conferences 2024 Asia
Piezoelectric Materials Conferences
Smart Materials Conferences 2024 USA
Artificial Materials Conferences
Smart Materials Conferences
Advanced Materials Conferences
Materials Science Conferences
Nanotechnology Conferences 2024
Smart Materials Conferences 2024
Nanotechnology Conferences 2024 Europe
Nanotechnology Conferences 2024 Asia
Smart materials, also known as smart or responsive materials, are materials that possess properties that can be altered in a controlled and reversible manner in response to external stimuli. These stimuli can include changes in temperature, light, pressure, electric or magnetic fields, pH, or the presence of specific substances. The advancements in smart materials have led to the development of innovative technologies and applications across various industries. Here are some key types of smart materials and their advancements:
Shape Memory Alloys (SMAs): SMAs, such as Nitinol (Nickel Titanium Naval Ordnance Laboratory), can return to a predetermined shape after deformation when subjected to a certain temperature change. Advances include the development of high-performance SMAs with improved mechanical properties and wider application ranges.
Piezoelectric Materials: Piezoelectric materials generate electric charge in response to mechanical stress and vice versa. Advancements in piezoelectric materials include the exploration of new compositions, flexible piezoelectric materials for wearable devices, and improved energy harvesting capabilities.
Electroactive Polymers (EAPs): EAPs can change their shape or size in response to an electric field. Developments in EAPs involve enhanced actuation performance, improved durability, and applications in soft robotics and haptic technology.
Shape-Changing Polymers: These polymers can change their shape or morphology in response to external stimuli. Advances include the design of stimuli-responsive polymers with applications in drug delivery, responsive textiles, and flexible electronics.
Smart Hydrogels: Hydrogels are water-absorbent polymers that can swell or shrink in response to changes in environmental conditions, such as pH, temperature, or the presence of specific ions. Smart hydrogels are utilized in drug delivery, tissue engineering, and responsive surfaces.
Magnetic Shape Memory Alloys (MSMAs): MSMAs can change their shape in response to a magnetic field. Advances involve the development of MSMAs with improved magnetic properties and applications in actuators and sensors.
Thermoelectric Materials: Thermoelectric materials convert temperature differences into electric voltage. Advances in thermoelectric materials include the discovery of new high-performance materials for waste heat recovery and energy harvesting.
Phase Change Materials (PCMs): PCMs can undergo a phase transition, such as melting or solidification, in response to changes in temperature. These materials are used for thermal energy storage and temperature regulation in buildings and electronic devices.
Responsive Nanomaterials: Nanomaterials, such as nanoparticles and nanocomposites, exhibit unique properties that can be manipulated by external stimuli. Advances involve the fabrication of responsive nanomaterials for applications in drug delivery, sensors, and nanoelectromechanical systems (NEMS).
Tags
Photovoltaic Materials Conferences
Nanotechnology Conferences 2024 Middle East
Advanced Materials Conferences
Nanotechnology Conferences 2024
Smart Materials Conferences 2024 USA
Smart Materials Conferences 2024 Japan
Smart Materials Conferences 2024 Aisa
Carbon Materials Conferences
Materials Science and Engineering Conferences
Nanotechnology Conferences 2024 USA
Materials Science Conferences 2024 Asia
Materials Science Conferences 2024 Middle East
Smart Materials Conferences 2024
Nanotechnology Conferences 2024 Europe
Materials Science Conferences 2024 Europe
Smart textiles, also known as smart fabrics or e-textiles, refer to textiles that are embedded with electronic components, sensors, or other advanced materials to impart additional functionalities. These textiles can respond to environmental stimuli, communicate data, or perform various tasks beyond the traditional roles of fabrics. Breakthroughs in smart textiles have led to innovations in wearable technology, healthcare, fashion, and other industries. Here are some key aspects and breakthroughs in the field of smart textiles:
Conductive Fabrics: The development of conductive textiles involves integrating conductive fibers or materials into fabrics, allowing them to transmit electrical signals. This breakthrough has paved the way for the creation of touch-sensitive fabrics, flexible circuits, and conductive yarns for various applications.
Wearable Sensors: Smart textiles incorporate sensors that can monitor physiological signals, such as heart rate, temperature, or motion. These sensors can be seamlessly integrated into clothing, enabling continuous health monitoring, sports performance tracking, and other personalized applications.
Thermochromic and Photochromic Textiles: Textiles with thermochromic properties change color in response to temperature variations, while photochromic textiles respond to light. Breakthroughs in these technologies have led to the creation of color-changing fabrics for fashion, sportswear, and outdoor applications.
Shape Memory Textiles: Shape memory textiles can change their shape or structure in response to external stimuli, such as temperature. This breakthrough enables the design of self-adjusting garments, smart orthopedic braces, and adaptive clothing for various purposes.
Flexible Displays: The development of flexible and stretchable displays allows for the incorporation of electronic displays into textiles. This breakthrough opens up possibilities for interactive clothing, dynamic patterns, and wearable information displays.
Textile-Based Electronics: Textile-based electronics involve the integration of electronic components, such as microcontrollers, batteries, and conductive threads, directly into textiles. This breakthrough enables the creation of smart garments with computational capabilities for various applications, including health monitoring and communication.
Responsive and Adaptive Fabrics: Responsive fabrics can react to changes in the environment, such as humidity or light, to provide enhanced comfort or functionality. Adaptive fabrics adjust their properties, such as porosity or insulation, based on external conditions.
Antimicrobial Textiles: Smart textiles with antimicrobial properties use materials that inhibit the growth of bacteria and other microorganisms. This breakthrough is particularly relevant in healthcare settings and for the development of odor-resistant sportswear.
Aesthetic and Interactive Fabrics: Breakthroughs in smart textiles include fabrics with aesthetic features, such as color-changing patterns or dynamic visual effects. Interactive textiles may respond to gestures or touch, providing a more engaging and personalized user experience.
Biometric Monitoring Textiles: Incorporating biometric monitoring capabilities into textiles allows for continuous tracking of vital signs and other physiological parameters. This breakthrough is valuable for applications in healthcare, fitness, and well-being.
Integration with Internet of Things (IoT): Smart textiles are increasingly being integrated into the Internet of Things (IoT) ecosystem. This allows for seamless connectivity and data exchange between textiles and other smart devices, enabling a more interconnected and intelligent environment.
Tags
Materials Science Conferences 2024 Middle East
Nanotechnology Conferences 2024 USA
Artificial Materials Conferences
Composite Materials Conferences
Photovoltaic Materials Conferences
Materials Science Conferences 2024 Europe
Piezoelectric Materials Conferences
Materials Science Conferences
Smart Materials Conferences
Materials Science Conferences 2024 USA
Smart Materials Conferences 2024 Aisa
Intelligent Materials Conferences
Smart Materials Conferences 2024
Materials Science Conferences 2024
Materials Science Conferences 2024 Asia
Smart windows with light-responsive properties, often referred to as dynamic or switchable windows, are windows that can change their optical properties in response to external stimuli such as light, heat, or an applied voltage. The goal is to control the amount of light, heat, and glare entering a building, optimizing energy efficiency and enhancing occupant comfort. Advancements in smart windows have led to innovations in architecture and building technologies. Here are key aspects and breakthroughs in the field:
Electrochromic Windows: Electrochromic windows can dynamically change their transparency or tint in response to an applied electric voltage. The electrochromic material absorbs or reflects light, allowing for the modulation of brightness and solar heat gain. Advances in electrochromic technology include improved switching speed, durability, and energy efficiency.
Photochromic Windows: Photochromic materials undergo reversible color changes in response to light exposure. Photochromic windows can darken when exposed to sunlight and revert to a clear state in the absence of light. Advancements involve the development of photochromic materials with enhanced responsiveness and longer lifetimes.
Thermochromic Windows: Thermochromic windows change their optical properties based on temperature variations. These windows can become darker in response to increased temperatures, reducing solar heat gain during warm periods. Breakthroughs include the design of thermochromic materials with precise control over their switching temperatures.
Suspended Particle Devices (SPDs): SPDs are windows that use suspended particles within a film to control light transmission. Applying an electric voltage causes the particles to align, allowing light to pass through. SPD windows offer dynamic control over glare and heat. Advances involve improved SPD technology, leading to faster switching times and better optical performance.
Liquid Crystal Windows: Liquid crystal windows utilize the properties of liquid crystals to modulate light transmission. An applied electric field can change the orientation of liquid crystal molecules, altering the transparency of the window. Advances include the development of liquid crystal materials with improved stability and performance.
Polymer Dispersed Liquid Crystal (PDLC) Windows: PDLC windows consist of liquid crystal droplets dispersed in a polymer matrix. When an electric field is applied, the droplets align, allowing light to pass through. PDLC windows offer privacy control and glare reduction. Breakthroughs involve advancements in the manufacturing process for improved reliability and optical quality.
Switchable Nanomaterials: Nanomaterials with switchable properties, such as nanoparticles or nanocrystals, are being explored for use in smart windows. These materials can change their optical characteristics in response to external stimuli, providing new avenues for developing dynamic window technologies.
Integration with Building Automation Systems: Smart windows are increasingly being integrated into building automation and control systems. This allows for coordinated control of lighting, HVAC, and shading systems based on real-time environmental conditions and user preferences.
Multifunctional Smart Windows: Advancements in materials science and nanotechnology have enabled the development of multifunctional smart windows that can perform multiple tasks simultaneously. For example, a smart window may not only control light transmission but also harvest energy and provide self-cleaning properties.
Tags
Materials Science and Engineering Conferences
Materials Science Conferences
Smart Materials Conferences
Smart Materials Conferences 2024 Japan
Piezoelectric Materials Conferences
Materials Science Conferences 2024 Europe
Smart Materials Conferences 2024 Aisa
Advanced Materials Conferences
Smart Materials Conferences 2024
Materials Science Conferences 2024
Smart Materials Conferences 2024 USA
Photovoltaic Materials Conferences
Nanotechnology Conferences 2024 Europe
Artificial Materials Conferences
Soft actuators are devices that can generate controlled and reversible movements or deformations while exhibiting flexibility and compliance. These actuators are often made from soft materials such as elastomers, hydrogels, or polymers, and they find applications in soft robotics, medical devices, wearable technologies, and various other fields. Breakthroughs in soft actuators have paved the way for the development of more versatile and adaptive technologies. Here are key aspects and breakthroughs in the field of soft actuators:
Pneumatic Actuators: Soft pneumatic actuators use pressurized air or other gases to drive their deformations. They are typically made of elastomeric materials and can exhibit a wide range of motions, including bending, twisting, and contracting. Breakthroughs in design and fabrication have led to more efficient and controllable pneumatic soft actuators.
Hydraulic Actuators: Similar to pneumatic actuators, hydraulic soft actuators use fluids to drive their movements. These actuators are often more compact than their pneumatic counterparts and can provide smoother and more controlled motions. Advances in materials and manufacturing techniques have improved the performance of hydraulic soft actuators.
Dielectric Elastomer Actuators (DEAs): DEAs are soft actuators that deform in response to an applied voltage. They are made from materials with electroactive properties, such as dielectric elastomers. Breakthroughs in DEA technology include improvements in the materials' performance, enhanced actuation capabilities, and the development of stretchable electrodes.
Shape Memory Polymers (SMPs): SMPs are soft materials that can "remember" a specific shape and return to it upon activation, often through changes in temperature. Soft actuators based on SMPs exhibit reversible deformations, making them suitable for various applications. Advances include the development of SMPs with tailored properties for specific uses.
Ionic Polymer-Metal Composite Actuators (IPMCs): IPMCs are soft actuators that respond to an applied electric field by bending or deforming. They are composed of a polymer matrix containing metal cations. Improvements in the design and fabrication of IPMCs have enhanced their performance in terms of speed, responsiveness, and durability.
Soft Robotics Actuators: Soft robotics involves the use of soft materials and compliant structures in robotic systems. Soft actuators play a crucial role in the field of soft robotics by providing the necessary flexibility and adaptability for tasks such as grasping, manipulation, and locomotion. Breakthroughs in soft robotics actuators include the development of novel designs, materials, and control strategies.
Bio-Inspired Soft Actuators: Breakthroughs in soft actuators include designs inspired by biological systems. For example, actuators mimicking the movements of muscles or tentacles have been developed. These bio-inspired soft actuators offer unique capabilities and are used in applications ranging from medical devices to soft grippers in robotics.
Stretchable and Wearable Actuators: Advancements in materials science and fabrication techniques have led to the development of stretchable and wearable soft actuators. These actuators can be integrated into wearable devices, providing comfortable and unobtrusive assistance or haptic feedback to users.
Soft Actuators for Rehabilitation Devices: Soft actuators have found applications in rehabilitation devices, assisting individuals with impaired mobility or motor control. Breakthroughs in this area include the development of soft exoskeletons and assistive devices that closely match the biomechanics of human movement.
Variable Stiffness Actuators: Soft actuators with variable stiffness properties can dynamically adjust their stiffness to adapt to different tasks or environmental conditions. This breakthrough is essential for applications where the ability to modulate stiffness is crucial, such as in robotic manipulation and grasping.
Tags
Materials Science Conferences
Materials Science Conferences 2024 Europe
Materials Science and Engineering Conferences
Smart Materials Conferences 2024 Europe
Smart Materials Conferences 2024 Aisa
Smart Materials Conferences 2024 USA
Nanotechnology Conferences 2024
Intelligent Materials Conferences
Smart Materials Conferences 2024
Nanotechnology Conferences 2024 Europe
Materials Science Conferences 2024 USA
Piezoelectric Materials Conferences
Smart Materials Conferences 2024 Japan
Artificial Materials Conferences
Composite Materials Conferences
Stimuli-responsive hydrogels are a class of materials that can undergo reversible changes in their volume, shape, or properties in response to specific external stimuli. These stimuli can include variations in temperature, pH, light, ionic strength, or the presence of certain molecules. Stimuli-responsive hydrogels have found applications in drug delivery, tissue engineering, sensors, and various biomedical and industrial fields. Here are key aspects and breakthroughs in the field of stimuli-responsive hydrogels:
Temperature-Responsive Hydrogels: These hydrogels undergo a reversible volume phase transition in response to changes in temperature. Advances include the development of thermoresponsive hydrogels with tunable lower and upper critical solution temperatures, enabling precise control over their swelling and deswelling behavior.
Light-Responsive Hydrogels: Light-responsive hydrogels undergo changes in their structure or properties when exposed to light of specific wavelengths. Photoresponsive groups, such as azobenzene or spiropyran, are incorporated into these hydrogels. Advances include improved photoresponsive materials and the development of light-triggered drug release systems.
Ionic Strength-Responsive Hydrogels: These hydrogels are sensitive to changes in the ionic strength of the surrounding environment. Ionic strength-responsive hydrogels are often employed in controlled drug release applications and biosensors. Breakthroughs involve optimizing the sensitivity and response range of these hydrogels.
Enzyme-Responsive Hydrogels: Hydrogels responsive to specific enzymes can undergo changes triggered by enzymatic reactions. These hydrogels have applications in biosensors and targeted drug delivery. Advances include the development of enzyme-specific recognition motifs for enhanced responsiveness.
Dual or Multi-Responsive Hydrogels: Breakthroughs in the field include the design of hydrogels that respond to multiple stimuli simultaneously. For example, a hydrogel may be responsive to both temperature and pH changes, offering more sophisticated and controllable behaviors.
Magnetic-Responsive Hydrogels: Incorporating magnetic nanoparticles or responsive polymers into hydrogels allows for manipulation using an external magnetic field. This feature is utilized in applications such as remotely controlled drug delivery and tissue engineering. Advances involve improving the magnetic responsiveness and biocompatibility of these hydrogels.
Mechanical Force-Responsive Hydrogels: Hydrogels that respond to mechanical forces, such as stretching or compression, have applications in soft robotics and wearable devices. Advances include the development of hydrogels with tailored mechanical properties and responsiveness to specific force thresholds.
Self-Healing Hydrogels: Self-healing hydrogels have the ability to repair damage autonomously. Breakthroughs involve incorporating dynamic chemical bonds or reversible physical interactions within the hydrogel structure, allowing it to recover from mechanical damage over time.
Injectable and Injectable Hydrogels: Advances in injectable hydrogels have facilitated minimally invasive delivery methods. Injectable hydrogels are often used in tissue engineering, wound healing, and drug delivery applications, offering precise and localized treatment.
3D Printing of Hydrogels: The integration of 3D printing techniques with hydrogel fabrication has enabled the creation of complex and customized structures for tissue engineering and drug delivery. This breakthrough allows for the precise control of hydrogel architecture and functionality.
Tags
Photovoltaic Materials Conferences
Nanotechnology Conferences 2024 Asia
Smart Materials Conferences 2024 Japan
Materials Science Conferences 2024 Middle East
Materials Science Conferences 2024 Europe
Carbon Materials Conferences
Artificial Materials Conferences
Piezoelectric Materials Conferences
Smart Materials Conferences 2024 Europe
Nanotechnology Conferences 2024 Europe
Materials Science Conferences
Smart Materials Conferences 2024
Nanotechnology Conferences 2024
Materials Science Conferences 2024
Materials Science Conferences 2024 Asia
Structural Health Monitoring (SHM) is a field of technology that involves the continuous monitoring and assessment of the structural condition of engineering structures, such as bridges, buildings, pipelines, and aerospace structures. The primary goal of SHM is to detect and assess damage, deterioration, or changes in the structural integrity of these systems, allowing for timely maintenance or intervention. Breakthroughs in SHM have been driven by advancements in sensor technologies, data analysis techniques, and the integration of smart materials. Here are key aspects and breakthroughs in the field of Structural Health Monitoring:
Sensor Technologies: Advances in sensor technologies play a crucial role in SHM. Traditional sensors like accelerometers, strain gauges, and displacement sensors have been complemented by newer and more sophisticated sensors, including fiber optic sensors, piezoelectric sensors, and wireless sensor networks. These sensors provide real-time data on structural responses, facilitating more accurate and comprehensive monitoring.
Wireless Sensor Networks: The use of wireless sensor networks has transformed the way structural health data is collected and transmitted. Wireless sensors offer the advantage of easy deployment, reduced wiring complexity, and the ability to monitor structures in real-time. This breakthrough has facilitated the development of large-scale and distributed SHM systems.
Data Fusion and Analytics: Breakthroughs in data fusion and analytics involve the integration of data from multiple sensors and sources to provide a holistic view of structural health. Advanced data analytics techniques, including machine learning and artificial intelligence, are employed to process and interpret the vast amount of data generated by sensors, enabling more accurate damage detection and prediction.
Non-Destructive Testing (NDT) Techniques: Non-destructive testing techniques, such as ultrasound, acoustic emission, and ground-penetrating radar, have seen advancements in terms of accuracy, resolution, and speed. These techniques allow for the inspection of structural components without causing damage, contributing to a more comprehensive assessment of the structural health.
Smart Materials and Embedded Sensors: The integration of smart materials, such as shape memory alloys and piezoelectric materials, into structures allows for the embedding of sensors directly into the material. This breakthrough enhances the sensitivity of SHM systems and enables more seamless integration with the structure itself.
Remote Sensing Technologies: Remote sensing technologies, including satellite imagery and unmanned aerial vehicles (UAVs), have been employed for large-scale structural monitoring. These technologies provide a broader perspective and are particularly useful for monitoring infrastructure in challenging or inaccessible environments.
Real-Time Monitoring and Alerts: The shift towards real-time monitoring allows for immediate detection of structural anomalies or damage. Breakthroughs in communication technologies enable real-time transmission of data to centralized monitoring systems, triggering alerts and enabling prompt decision-making regarding maintenance or intervention.
Integration with Building Information Modeling (BIM): Integrating SHM data with Building Information Modeling enhances the understanding of a structure's performance and health. This integration allows for a more holistic approach to infrastructure management, combining data from design, construction, and operation phases.
Smart Infrastructure Development: Cities and infrastructure are becoming "smart" with the integration of SHM technologies. Smart infrastructure can adapt to changing conditions, optimize resource use, and enhance overall resilience. This breakthrough is particularly relevant in the context of developing sustainable and resilient urban environments.
Innovations in Materials and Coatings: Advances in materials science have led to the development of coatings and materials with built-in sensing capabilities. These materials can act as sensors themselves, providing continuous monitoring of structural health while minimizing the need for external sensors.
Tags
Materials Science Conferences 2024
Artificial Materials Conferences
Composite Materials Conferences
Materials Science Conferences 2024 Middle East
Smart Materials Conferences 2024
Nanotechnology Conferences 2024 Asia
Photovoltaic Materials Conferences
Smart Materials Conferences
Nanotechnology Conferences 2024
Carbon Materials Conferences
Advanced Materials Conferences
Nanotechnology Conferences 2024 Europe
Materials Science Conferences 2024 Europe
Smart Materials Conferences 2024 Aisa
Intelligent Materials Conferences
Thermochromic materials are substances that undergo a reversible change in color or transparency in response to changes in temperature. These materials are often used in various applications, including smart windows, textiles, packaging, and temperature indicators. The advancement of thermochromic materials has been driven by research in materials science, chemistry, and engineering, leading to improvements in performance, versatility, and commercial viability. Here are key aspects and advancements in the field of thermochromic materials:
Smart Windows: Thermochromic materials are extensively used in smart windows that can dynamically control the transmission of light and heat. When the temperature changes, these materials alter their color or transparency, allowing for the regulation of solar heat gain and glare. Advanced thermochromic windows contribute to energy efficiency in buildings by optimizing natural lighting and reducing the need for artificial heating or cooling.
Responsive Textiles: Thermochromic materials are incorporated into fabrics to create textiles that change color with temperature variations. This application is popular in fashion, sportswear, and outdoor gear, providing both aesthetic appeal and functional benefits. For example, color-changing clothing can indicate temperature changes or serve as a design element.
Temperature-Indicating Labels and Inks: Thermochromic inks and labels are widely used as temperature indicators on various products, such as beverage containers, baby bottles, and promotional items. The color change signals the temperature of the contents, providing a visual cue for users. Advances in this area include improved ink formulations for durability and accuracy.
Packaging Materials: Thermochromic materials are applied to packaging materials to indicate temperature changes during transportation or storage. This helps in monitoring the integrity of temperature-sensitive products, such as food, pharmaceuticals, and sensitive electronic components. Advancements involve the development of cost-effective and scalable thermochromic packaging solutions.
Inkjet Printing Technology: Advances in inkjet printing technology have facilitated the precise deposition of thermochromic inks on various surfaces. This allows for the creation of detailed and customizable designs, patterns, or messages that become visible or change color at specific temperature thresholds.
Nanotechnology Integration: Nanotechnology has played a role in advancing thermochromic materials. Nanoparticles and nanocomposites are used to enhance the performance of thermochromic coatings, offering improved stability, response times, and color options. Nanomaterials also contribute to the development of thin and flexible thermochromic films.
Multi-Color Thermochromic Systems: Recent advancements include the development of thermochromic materials capable of exhibiting multiple colors at different temperature ranges. This allows for a more nuanced representation of temperature changes and expands the potential applications in visual displays and aesthetic designs.
High-Performance Pigments: Research has led to the discovery and development of high-performance pigments with superior thermochromic properties. These pigments exhibit vivid color changes, high sensitivity to temperature variations, and improved durability under different environmental conditions.
Adaptive Camouflage: Thermochromic materials are explored for applications in adaptive camouflage systems. These materials can change color to match the surrounding temperature, providing potential applications in military and defense for thermal concealment.
Integration with Wearable Devices: Thermochromic materials are integrated into wearable devices, such as smart textiles and accessories, to add visual and temperature-responsive elements. These applications contribute to the development of interactive and personalized wearable technologies.
Biomedical Sensors: Thermochromic materials are explored for biomedical applications, such as in the development of sensors that can indicate changes in temperature within the body. These sensors have potential applications in medical diagnostics and monitoring.
Tags
Nanotechnology Conferences 2024 Middle East
Smart Materials Conferences 2024 Aisa
Piezoelectric Materials Conferences
Smart Materials Conferences 2024 Europe
Photovoltaic Materials Conferences
Materials Science Conferences 2024 Middle East
Materials Science and Engineering Conferences
Materials Science Conferences 2024
Artificial Materials Conferences
Smart Materials Conferences 2024 USA
Nanotechnology Conferences 2024 Europe
Nanotechnology Conferences 2024
Composite Materials Conferences
Materials Science Conferences 2024 Asia
Carbon Materials Conferences
Wearable healthcare devices are electronic devices that are worn on the body and designed to monitor, track, and manage various aspects of health and well-being. These devices leverage sensors, connectivity, and data analysis to provide users and healthcare professionals with valuable insights into physiological parameters, activity levels, and health metrics. Breakthroughs in wearable healthcare devices have been transformative, enabling more personalized and proactive healthcare monitoring. Here are key aspects and breakthroughs in this field:
Biometric Sensors: Wearable devices incorporate a variety of biometric sensors to measure parameters such as heart rate, blood pressure, body temperature, electrocardiogram (ECG), and more. Advances in sensor technology have improved accuracy, sensitivity, and reliability, making these devices valuable for health monitoring.
Activity Tracking and Motion Sensors: Wearable devices equipped with accelerometers and motion sensors can track physical activity, count steps, and monitor movement patterns. This is essential for assessing overall activity levels, encouraging physical fitness, and identifying potential health issues.
Continuous Glucose Monitoring (CGM): CGM devices are a breakthrough for individuals with diabetes, allowing continuous monitoring of glucose levels throughout the day. These devices provide real-time data, reducing the need for frequent fingerstick tests and enabling more precise insulin management.
Advanced Sleep Monitoring: Wearable devices with advanced sleep monitoring capabilities track sleep patterns, duration, and quality. Breakthroughs include the development of devices that can provide insights into sleep stages, detect sleep disorders, and offer personalized recommendations for better sleep hygiene.
Remote Patient Monitoring (RPM): RPM systems use wearable devices to remotely monitor patients' health conditions. This is particularly beneficial for individuals with chronic illnesses or those recovering from surgeries. Breakthroughs in RPM have improved the accuracy of data transmission, facilitating timely interventions and reducing hospital readmissions.
Smart Fabrics and Textiles: The integration of sensors into smart fabrics and textiles has expanded the possibilities for wearable devices. Breakthroughs include clothing with embedded sensors for monitoring vital signs, providing a more seamless and unobtrusive monitoring experience.
Wearable ECG Monitors: Wearable electrocardiogram (ECG) monitors have become more sophisticated, offering continuous monitoring of heart rhythm and early detection of irregularities. Some devices can even generate medical-grade ECG reports that can be shared with healthcare professionals.
Telemedicine Integration: Wearable devices are increasingly integrated with telemedicine platforms, allowing real-time sharing of health data with healthcare professionals. This breakthrough has facilitated remote consultations, proactive care, and improved healthcare accessibility.
Artificial Intelligence (AI) and Machine Learning: The integration of AI and machine learning algorithms has enhanced the capabilities of wearable devices for data analysis and pattern recognition. This allows for more accurate and personalized health insights, early disease detection, and tailored recommendations.
Wearable Drug Delivery Systems: Some wearable devices incorporate drug delivery mechanisms, allowing for the continuous and controlled administration of medication. This is particularly relevant in conditions that require precise and timely drug dosing.
Emotional and Mental Health Monitoring: Breakthroughs in wearable technology include devices designed to monitor emotional and mental well-being. These devices may incorporate sensors to detect stress levels, assess mood, or provide feedback for relaxation techniques.
Customization and User Engagement: Wearable healthcare devices are becoming more customizable to individual preferences, allowing users to tailor their experience. Gamification and interactive features contribute to increased user engagement, encouraging adherence to health and wellness routines.
The continuous evolution of wearable healthcare devices involves interdisciplinary collaboration, incorporating insights from materials science, electronics, data science, and healthcare. As these devices become more sophisticated, they play a pivotal role in preventive care, early detection, and patient-centered healthcare management.
Tags
Nanotechnology Conferences 2024 Middle East
Piezoelectric Materials Conferences
Artificial Materials Conferences
Materials Science Conferences 2024 Europe
Smart Materials Conferences 2024 Japan
Smart Materials Conferences
Photovoltaic Materials Conferences
Smart Materials Conferences 2024 Europe
Materials Science Conferences
Intelligent Materials Conferences
Materials Science Conferences 2024 USA
Composite Materials Conferences
Smart Materials Conferences 2024 USA
Smart Materials Conferences 2024
Smart Materials Conferences 2024 Aisa
Market Analysis:
Global Smart Materials-Market Insights:
The Advanced Smart Materials Market is on track to reach a remarkable $2.1 trillion by 2025, with a robust compound annual growth rate (CAGR) of 4.5% from 2020 to 2025. This surge is revolutionizing the global manufacturing sector, particularly notable for its role in displacing traditional plastics and metals with cutting-edge ceramics and composites in high-performance applications. This transformation is driven by a growing public interest in seeking alternatives for end products, contributing to the escalating demand for advanced materials throughout the forecast period.
The global polymer market, valued at $533.6 billion in 2019, is projected to experience a substantial CAGR of 5.1% from 2020 to 2030, ultimately reaching $838.5 billion. The driving force behind this growth is the surging demand for polymers in crucial industries such as packaging, automotive, and electronics. Plastics, a subset of polymers, are especially in high demand in the packaging sector due to their advantageous characteristics.
Simultaneously, the global biomaterials market is poised for substantial growth, increasing from USD 35.5 billion in 2020 to USD 47.5 billion by 2025, reflecting a CAGR of 6.0%. This growth is fueled by various factors, including increased funding and grants from government bodies worldwide to support the development of novel biomaterials. The rising demand for medical implants, coupled with heightened research efforts in regenerative medicine, further contributes to the market's expansion. Additionally, the growing incidence of cardiovascular diseases adds momentum to the overall growth trajectory of the biomaterials market
Market Insights of Smart Materials in USA:
The global smart materials market was valued at USD 58.95 billion in 2022, increasing at a CAGR of 13.04% from 2023 to 2032 and anticipated to reach USD 200.82 billion by 2032. Also, the rising demand from key verticals like aerospace and consumer electronics will drive market growth during the forecast period.
Moreover, the technological advancements and innovative product launches by manufacturers, along with increasing demand for smart packaging in consumer goods, are also helping to boost the market growth during the forecast period.
Market Insights of Smart Materials in Europe:
Europe is the second major exporting region for Smart Materials due to its capacity. The European market is poised for steady revenue growth in the forecast period, driven by the burgeoning development of the automotive industry in key countries like the U.K., Germany, and France. The robust expansion of the automotive sector fuels a heightened demand for smart materials across Europe. Notably, Europe serves as a significant hub for automotive giants such as Audi AG, Volkswagen Group, Fiat Chrysler Automobiles N.V., among others.
Europe held a market share of about 22.5% in the Smart Material Market in 2023 and is expected CAGR 6.9% to maintain its dominance till 2030. Increasing demand for electric, plug-in, and hybrid electric vehicles, so smart material market development is increasing in the future.
Additionally, the rising adoption of smart sensors in medical applications is anticipated to contribute to the revenue growth of the market in Europe, further accentuating its potential for expansion.
Market Insights of Smart Materials in Acia Pacific:
As of the latest analysis from Meticulous Research, the smart materials in the Asia-Pacific region are poised to reach a value of $120.8 billion by 2030, demonstrating a robust Compound Annual Growth Rate (CAGR) of 16.2% from 2023 to 2030. This upward trajectory is primarily attributed to factors such as the increasing pace of urbanization, elevated income levels in developing nations, government initiatives promoting the adoption of smart technology, and a growing emphasis on enhancing home energy efficiency.
The market is further propelled by the rising demand for personalized and adaptable home appliances and devices that cater to individual consumer preferences, presenting substantial growth opportunities.
The Asia-Pacific smart materials market is systematically categorized based on product type and protocols & standards. The comprehensive report not only delves into an in-depth analysis of industry competitors but also provides insights into market dynamics at both regional and country levels.
Market Insights of Smart Materials in Middle-East:
The Smart Materials Market in the Middle East & Africa market is projected to reach $40.38 billion by 2030, at a CAGR of 25% during the forecast period. These smart materials, characterized by their controlled responsiveness to changing environments, demonstrate unique molecular structures that react to external stimuli such as electric fields, magnetic fields, pressure, temperature, moisture, and chemicals.
The escalating demand for automated and sophisticated machinery and devices among modern consumers has been a key driver for the increased adoption of piezoelectric devices. This trend is further fueled by the imperative to promote the utilization of non-conventional energy sources.
Global Energy Materials -Market Insights:
Global Advanced Energy Materials Market is estimated to be worth USD 1.90 Billion in 2022, if current trends persist, projections indicate that by 2040, global energy consumption will escalate to a staggering 740 million terajoules, marking a significant 30% increase. Over the span of two decades, from 2000 to 2040, this trajectory translates to a substantial 77% rise in global energy consumption.
Taking a broader perspective, from 1980 to 2050, experts forecast that global energy usage could triple, soaring from approximately 300 to 900 million terajoules. Such figures underscore the monumental scale of energy consumption on a global scale, reflecting the profound impact on resources and environmental sustainability.
Market Insights of Energy Materials in USA:
The U.S. Energy Market exhibited robust expansion, surpassing a valuation of USD 68.6 billion in 2023, with a projected Compound Annual Growth Rate (CAGR) of 15.5% from 2024 to 2032. This noteworthy growth trajectory is primarily attributed to the escalating demand for the refurbishment and modernization of the existing grid network in the United States.
The surge in demand for reliable grid support mechanisms, coupled with an increasing integration of clean energy technologies, is steering the energy market towards significant development. The ongoing investments in infrastructure and a substantial rise in electricity demand further contribute to the overall expansion of the industry. Moreover, the landscape is enriched by technological innovations dedicated to enhancing energy efficiency, accompanied by a notable increase in renewable storage capacity.
As the industry continues to evolve, these factors collectively create a favorable and dynamic business environment, promising continued growth and innovation in the U.S. Energy Market in the years to come.
Market Insights of Energy Materials in Europe:
The Europe thermal energy storage market is projected to experience growth, increasing from US$ 7,183.01 million in 2022 to US$ 10,497.06 million by 2030, with an estimated Compound Annual Growth Rate (CAGR) of 4.9% between 2022 and 2030.
The market is categorized into various segments, including technology, storage material, application, end user, and country. In terms of technology, it is divided into sensible heat storage, latent heat storage, and thermochemical storage, with the sensible heat storage segment holding the largest market share in 2022.
Market Insights of Energy Materials in Acia Pacific:
The Asia Pacific Energy Market, valued at US$ 488.85 billion in 2023, is positioned for substantial growth at a CAGR of 9.73%.
The Asia-Pacific energy materials market is expected to grow with a CAGR of 4.85% during the forecast period, 2022-2030, and is projected to reach $65.38 billion in revenue by 2030.
This robust expansion is driven by the region's significant population share, accounting for 60% of the global population. The surge in energy demand within the Asia Pacific outpaces that of North America and Europe.
Particularly noteworthy is the region's leadership in renewable energy output, with major players like Australia, China, India, and Japan investing significantly in their renewable sectors.
The region is undergoing rapid urbanization and industrialization, leading to a substantial increase in energy demand. To address long-term sustainability concerns, there is a growing necessity to shift away from carbon-based technologies.
Several nations across the Asia Pacific region are actively unveiling power development plans, signifying a collective effort to embrace renewable energy during the forecast period.
Market Insights of Energy Materials in Middle-East:
The Middle East & Africa Materials energy Market is projected to reach a value of US$ 23,755.36 million by 2030, with a Compound Annual Growth Rate (CAGR) of 9.0%, according to Business Market Insights.
In the medium term, the market is anticipated to be primarily driven by governmental initiatives aimed at diversifying the energy mix and fostering increased investments in renewable energy.
The United Arab Emirates (UAE) stands out as a key player in the regional market, expected to experience significant growth during the forecast period. This growth is attributed to upcoming projects and ambitious government targets aimed at propelling the nation into a leading position within the Middle Eastern renewable energy sector. The UAE's commitment to sustainable energy practices and its proactive approach to renewable projects position it as a significant contributor to the overall growth and development of the regional renewable energy market.
Top 100 Smart Material Science Companies in Europe:
2D Fab, Switzerland / Acciona, Spain / Adama Innovations, United Kingdom / Advano, France / AGC Glass Europe, Belgium / AGC Plasma Technology Solutions Belgium / Aixtron SE, Germany / Anovion Battery Materials, Belgium / Applied Nanodetectors, United Kingdom / Aquafil Group, Italy / Archimedes IP, United Kingdom / Arkema, France / Attolight AG, Switzerland / Axcentive SARL, France / Belectric, Germany / BeNano Health, United Kingdom / Bioelektra Group, Poland / Bluon Energy, United Kingdom / Borit NV, Belgium / Calix Limited, United Kingdom / Cambridge Nanosystems, United Kingdom / Carbon Waters, France / CDT, United Kingdom / CEA Tech, France / CelluComp Ltd, United Kingdom / Ceramaret SA, Switzerland / CeramTec, Germany / Ceres Nanosciences, United Kingdom / Ceres Power, United Kingdom / CIC nanoGUNE, Spain / Coolrec, Netherlands / Covestro AG, Germany / Cytosurge AG, Switzerland / DSM, Netherlands / Dynamit Nobel Kunststoff GmbH Germany / Econcore NV, Belgium / EeStairs Netherlands / Elkem Silicones, Norway / ElringKlinger AG, Germany / Epishine, Sweden / Evonik Industries, Germany / Front Edge Technology, Sweden / G24 Power Ltd, United Kingdom / Gait Up, Switzerland / Graphene 3D Lab, United Kingdom / Graphene Batteries AS, Norway / Graphene Labs, Switzerland / Graphene Lighting plc United Kingdom / Graphene Square Inc., Switzerland / Graphenea Nanomaterials, Spain / Graphenea, Spain / Graphensic AB, Sweden / Graphitene, United Kingdom / Graphmatech, Sweden / Haydale Graphene Industries, United Kingdom / Heraeus Holding GmbH, Germany / Ilika Technologies, United Kingdom / IMEC, Belgium / Innovalight Germany / Integrated Graphene Netherlands / Italmatch Chemicals Italy / Johnson Matthey, United Kingdom / Keronite, United Kingdom / Leoni AG, Germany / LiquiGlide Inc., United Kingdom / Luxexcel, Netherlands / Materia Nova, Belgium / Merck Group, Germany / M-Solv Ltd, United Kingdom / Nanoco Group plc, United Kingdom / Nanoscribe GmbH, Germany / Nantero, Ireland / Neaspec GmbH, Germany / Nouryon, Netherlands / Novaled GmbH, Germany / OCSiAl, Luxembourg Oxford Advanced Surfaces Group plc, United Kingdom / Paragraf, United Kingdom / Pireta Limited United Kingdom / Plansee Group, Austria / PolyPhotonix, United Kingdom / SAES Group, Italy / Saint-Gobain, France / SGL Carbon, Germany / Siemens AG, Germany / SilcoTek Corporation, United Kingdom / Sixonia Tech, Germany / Solvay, Belgium / Synthomer, United Kingdom / Thales Group, France / TNO, Netherlands Organization for Applied Scientific Research / Trelleborg AB, Sweden / Varta AG, Germany / Versarien plc, United Kingdom / Voxeljet AG, Germany / XG Sciences, United Kingdom / Zinergy UK Ltd, United Kingdom / ZSW, Germany
Top 100 Smart Materials Companies in USA:
3M / A. Schulman, Inc. / Aculon Inc. / Aerogel Technologies / Aerogel Technologies / AGC Plasma Technology Solutions / Alchemy Mineral LLC / ALD Nanosolutions Inc. / Aledia / ALTAIR Nanotechnologies Inc. / American Elements / American Superconductor Corporation / Arkema / Arkema SA / Atomera / Avery Dennison Corporation / BASF / Bayer AG / Bcomp Ltd. / Blue Current / Blue Spark Technologies / Bluon Energy / Brewer Science / C3Nano / Cadenza Innovation / Cambrios Advanced Materials Corporation / Cambrios Technologies Corporation / Carbon Waters / Carpenter Technology Corporation / Celanese Corporation / Cima Nanotech / Contour Energy Systems / CoreTech System Co., Ltd. / Corning Incorporated / Cytec Solvay Group / Daikin Industries, Ltd. / Dow Chemical Company / DuPont / Eastman Chemical Company / Echogen Power Systems / Eikos Inc. / Enevate Corporation / Entegris, Inc. / Evonik Industries / Evraz Highveld Steel and Vanadium Limited / Ferro Corporation / General Electric / General Nano LLC / Gentex Corporation / Graphene Energy Inc. / Graphene Frontiers / Henkel AG & Co. KGaA / Hitachi Chemical Company / Honeywell International Inc. / Huntsman Corporation / J.M. Huber Corporation / Johnson Controls / Kennametal Inc. / Kuraray Co., Ltd. / Lanxess AG / Liquid Piston / Materion Corporation / Mitsubishi Chemical Corporation / Molecular Glasses Inc. / Molecular Imprints / Molecular Rebar Design / Momentive Performance Materials Inc. / Morgan Advanced Materials plc / MultiMechanics / NanoGraf Corporation / Nanoramic Laboratories / NBD Nanotechnologies Inc. / Novasentis / Novomer Inc. / Nth Degree Technologies Worldwide Inc. / Owens Corning / Pellion Technologies / PneumatiCoat Technologies / PPG Industries / Printechnologics / QD Vision Inc. / Raydiance / Rogers Corporation / SABIC / Saint-Gobain / Sekisui Chemical Co., Ltd. / Showa Denko K.K. / Sila Nanotechnologies / Silatronix / Silicor Materials / SmartKem / Solantro Semiconductor / Solvay / Sporian Microsystems / Sumitomo Chemical Co., Ltd. / Surface Generation / Teijin Limited / ThruWave / Toray Industries, Inc. / Trelleborg AB / Wacker Chemie AG / Zircar Ceramics Inc. / Zymergen
Top 100 Smart Materials Companies in Asia:
Amorepacific Corporation, South Korea / Asahi Kasei Corporation, Japan / BOE Technology Group Co., Ltd., China / Bridgestone Corporation, Japan / Bridgestone Corporation, Japan / BYD Company Limited, China / Canon Inc., Japan / Aerospace Science and Industry Corporation, China / Baowu Steel Group Corporation Limited, China / Communications Construction Company Limited, China / COSCO Shipping Corporation Limited, China / Electronics Corporation, China / Energy Investment Corporation, China / Everbright Group, China / General Nuclear Power Group, China / General Technology, China / Minmetals Corporation, China / China National Building Material Group Co. Ltd., China / National Chemical Corporation, China / National Nuclear Corporation, China / China National Offshore Oil Corporation, China / China North Industries Group Corporation Limited, China / China Railway Construction Corporation Limited, China / China Satellite Communications Co. Ltd., China / China Shipbuilding Industry Corporation, China / China South Industries Group Corporation, China / China State Construction Engineering Corporation, China / China Three Gorges Corporation, China / Daikin Industries, Japan / Denso Corporation, Japan / Doosan Corporation, South Korea / Doosan Heavy Industries & Construction, South Korea / Doosan Heavy Industries & Construction, South Korea / Foxconn Technology Group, Taiwan / Fujifilm Holdings Corporation, Japan / GS Engineering & Construction Corp., South Korea / Hankook Tire & Technology Co. Ltd., South Korea / Hankook Tire & Technology, South Korea / Hanwha Aerospace, South Korea / Hanwha Chemical Corporation, South Korea / Hanwha Corporation, South Korea / Hanwha Q CELLS, South Korea / Hanwha Solutions, South Korea / Hitachi Ltd., Japan / Hyosung Corporation, South Korea / Hyundai Engineering & Construction, South Korea / Hyundai Motor Company, South Korea / Kolon Industries, South Korea / Kyocera Corporation, Japan / LG Chem, South Korea / LG Display, South Korea / LG Hausys, South Korea / LG Innotek, South Korea / Lotte Chemical, South Korea / LS Cable & System Ltd., South Korea / Luxshare Precision Industry Co. Ltd., China / Mitsubishi Electric Corporation, Japan / Mitsui Chemicals Inc., Japan / Murata Manufacturing Co. Ltd., Japan / NEC Corporation, Japan / Neuronano Co. Ltd., South Korea / Nippon Electric Glass Co. Ltd., Japan / Nippon Steel Corporation, Japan / Nippon Steel Engineering Co. Ltd., Japan / Nitto Denko Corporation, Japan / OCI Company Ltd., South Korea / Olympus Corporation, Japan / Panasonic Corporation, Japan / POSCO, South Korea / Samsung Advanced Institute of Technology, South Korea / Samsung Display, South Korea / Samsung Electro-Mechanics, South Korea / Samsung Engineering, South Korea / SAMSUNG SDI CO. LTD., South Korea / Samyang Corporation, South Korea / Sekisui Chemical Co. Ltd., Japan / Showa Denko K.K., Japan / Sinochem Group Co. Ltd., China / SK Hynix, South Korea / SK Innovation, South Korea / SK Networks Co. Ltd., South Korea / SKC, South Korea / Sony Corporation, Japan / Sumitomo Chemical Co. Ltd., Japan / Sumitomo Electric Industries, Ltd., Japan / Teijin Limited, Japan / Toray Advanced Materials Korea Inc., South Korea / Toray Industries, Inc., Japan / Toshiba Corporation, Japan / Toyota Motor Corporation, Japan / Wanhua Chemical Group Co. Ltd., China
Top 100 Smart Materials Companies in Middle-East:
Abu Dhabi National Chemicals Company, UAE / Abu Dhabi National Oil Company, UAE / Abu Dhabi Polymers Company Ltd., UAE / Advanced Metal Industries Cluster Company, Bahrain / Advanced Petrochemical Company, Saudi Arabia / Advanced Water Technology, UAE / Ajmal Steel Tubes and Pipes Industries LLC, UAE / Al Bader International Development Company, Bahrain / Al Bawani Co. Ltd., Saudi Arabia / Al Gharbia Pipe Company, UAE / Al Muftah Group, Qatar / Al Nasser Industrial Enterprises LLC, UAE / Al Reyami Steel Construction Factory, UAE / Al Saghyir Boilers & Pressure Vessels Factory Co. Ltd., Saudi Arabia / Al Yamamah Steel Industries Co., Saudi Arabia / Alba Aluminium Bahrain, Bahrain / Al-Babtain Power & Telecommunication Co., Kuwait / Alcoa, UAE / Aluminium Bahrain, Bahrain / Arabian Cement Company, Saudi Arabia / Arabian Cement Industry LLC, UAE / Arabian Fiberglass Insulation Company Ltd., Saudi Arabia / Arabian Gulf Steel Industries LLC, UAE / Arabian Pipes Company, Saudi Arabia / Aramco, Saudi Arabia / Aramco Overseas Company, Saudi Arabia / Astra Industrial Group, Saudi Arabia / Bahrain Petroleum Company, Bahrain / Borouge, UAE / Dana Gas, UAE / Dolphin Energy, UAE / Dragon Oil, UAE / Dubai Aluminum, UAE / Dubai Cable Company, UAE / Emirates Extrusion Factory, UAE / Emirates Float Glass, UAE / Emirates Global Aluminium, UAE / Emirates Industrial Panel, UAE / Emirates Nuclear Energy Corporation, UAE / Emirates Steel Industries, UAE / EQUATE Petrochemical Company, Kuwait / Fajr Petrochemical Company, Iran / Global Gypsum Co. LLC, UAE / Gulf Cement Company, UAE / Gulf Cement Company, Saudi Arabia / Gulf Energy Maritime, UAE / Gulf Glass Industries, UAE / Gulf International Chemicals, Saudi Arabia / Gulf Petrochemical Industries Company, Bahrain / Gulf Petrochemicals and Chemicals Association, UAE / Industries Qatar, Qatar / International Maritime Industries, Saudi Arabia / International Petroleum Investment Company, UAE / Kuwait Foreign Petroleum Exploration Company, Kuwait / Kuwait Foundry Co., Kuwait / Kuwait Gulf Oil Company, Kuwait / Kuwait Petroleum Corporation, Kuwait / Kuwait Styrene Company, Kuwait / Ma’aden - Saudi Arabian Mining Company, Saudi Arabia / Mubadala Development Company, UAE / National Aluminium Products Company, Saudi Arabia / National Company for Glass Industries, Saudi Arabia / National Industrialization Company, Saudi Arabia / National Iranian Oil Refining and Distribution Company, Iran / National Iranian Petrochemical Company, Iran / National Metal Manufacturing and Casting Co., Saudi Arabia / National Petrochemical Company, Iran / National Petrochemical Industrial Company, Saudi Arabia / National Pipe Company Ltd., Saudi Arabia / Nesma Holding Co. Ltd., Saudi Arabia / Oman LNG, Oman / Oman Oil Company - Oman / Oman Oil Refineries and Petroleum Industries Company, Oman / Orpic-Oman Oil Refineries and Petroleum Industries Company, Oman / Petro Rabigh, Saudi Arabia / Petrochemical Conversion Company Ltd., Saudi Arabia / Petrochemical Industries Company, Kuwait / Qatar Chemical Company, Qatar / Qatar Fertiliser Company, Qatar / Qatar Fuel, Qatar / Qatar International Petroleum Marketing Company Ltd., Qatar / Qatar Petrochemical Company, Qatar / Qatar Steel, Qatar / Qatar Vinyl Company, Qatar / Qurain Petrochemical Industries Company, Kuwait / RAK Ceramics, UAE / Red Sea Housing Services, Saudi Arabia / Salalah Methanol Company LLC, Oman / Saudi Arabian Amiantit Company, Saudi Arabia / Saudi Arabian Fertilizer Company, Saudi Arabia / Saudi Arabian Mining Company, Saudi Arabia / Saudi Arabian Oil Company, Saudi Arabia / Saudi Basic Industries Corporation, Saudi Arabia / Saudi Cement Company, Saudi Arabia / Saudi International Pipe Coating Company, Saudi Arabia / Sipchem, Saudi Arabia / Takreer, UAE / The National Titanium Dioxide Company Ltd., Saudi Arabia / Union Pipes Industry, UAE / United Gulf Steel Mill Co. Ltd., Saudi Arabia / United Metal Coating LLC, UAE / United Steel Industrial Company, UAE / Yanbu National Petrochemical Company, Saudi Arabia
Top 100 Smart Materials Journals:
Nature Materials / ACS Applied Materials & Interfaces / ACS Nano / Acta Biomaterialia / Acta Crystallographica Section B: Structural Science, Crystal Engineering and Materials / Acta Materialia / Advanced Energy Materials / Advanced Functional Materials / Advanced Materials / Advanced Materials Interfaces / Applied Physics Letters / Biomacromolecules / Biomaterials / Carbon / Cement and Concrete / Research / Chemical Communications / Chemistry of Materials / Computational Materials Science / Corrosion Science / Electrochimica Acta / Energy & Environmental Science / IEEE Transactions on Nanotechnology / International Journal of Biological Macromolecules / International Journal of Hydrogen Energy / International Journal of Pharmaceutics / International Journal of Refractory Metals and Hard Materials / Journal of Advanced Ceramics / Journal of Alloys and Compounds / Journal of Applied Electrochemistry / Journal of Applied Physics / Journal of Biomedical Materials Research Part A / Journal of Biomedical Materials Research Part B: Applied Biomaterials / Journal of Coatings Technology and Research / Journal of Colloid and Interface Science / Journal of Composite Materials / Journal of Composite Materials / Journal of Crystal Growth / Journal of Hazardous Materials / Journal of Inorganic and Organometallic Polymers and Materials / Journal of Magnetism and Magnetic Materials / Journal of Materials Chemistry A / Journal of Materials Processing Technology / Journal of Materials Research / Journal of Materials Science / Journal of Materials Science & Technology / Journal of Materials Science: Materials for a Sustainable Future / Journal of Materials Science: Materials for Advanced Technologies / Journal of Materials Science: Materials for Electronic and Optical Devices / Journal of Materials Science: Materials for Electronics / Journal of Materials Science: Materials for Electronics and Optoelectronics / Journal of Materials Science: Materials for Energy Systems / Journal of Materials Science: Materials for Green Energy / Journal of Materials Science: Materials for Infrastructure / Journal of Materials Science: Materials for Medicine / Journal of Materials Science: Materials in Medicine / Journal of Nanoparticle Research / Journal of Physical Chemistry Letters / Journal of Polymer Science Part B: Polymer Physics / Journal of Power Sources / Journal of Reinforced Plastics and Composites / Journal of Sol-Gel Science and Technology / Journal of Solid State Chemistry / Journal of the American Society for Mass Spectrometry / Journal of the Mechanical Behavior of Biomedical Materials / Journal of the Society of Materials Science, Japan / Journal of Vacuum Science & Technology B / Langmuir / Materials and Design / Materials Chemistry and Physics / Materials Horizons / Materials Letters / Materials Research Bulletin / Materials Science and Engineering: R: Reports / Materials Science in Semiconductor Processing / Materials Today / Materials Today Physics / Nano Letters / Nano Today / Physical Review Letters / Polymer / Polymer Degradation and Stability / Progress in Materials Science / Progress in Polymer Science / Science Advances / Scripta Materialia / Smart Materials and Structures / Surface Science Reports / Thin Solid Films
Smart Material Societies in USA:
Materials Research Society / American Ceramic Society / American Chemical Society / American Concrete Institute / American Gear Manufacturers Association / American Institute for Medical and Biological Engineering / American Institute of Chemical Engineers / American Physical Society / American Physical Society Division of Materials Physics / American Society for Composites / American Society for Engineering Education / American Society for Metals International / American Society for Microbiology / American Society for Nondestructive Testing / American Society for Precision Engineering / American Society for Precision Engineering / American Society for Testing and Materials / American Society of Biomechanics / American Society of Civil Engineers / American Society of Heating, Refrigerating and Air-Conditioning Engineers / American Society of Mechanical Engineers / American Society of Safety Professionals / American Vacuum Society / American Welding Society / Association for Iron & Steel Technology / Electrochemical Society / Federation of Asian Chemical Societies / Federation of European Materials Societies / Federation of Materials Societies / Institute of Electrical and Electronics Engineers / Institute of Materials, Minerals and Mining / International Association for Structural Mechanics in Reactor Technology / International Association of Advanced Materials / International Organization on Shape Memory and Super elastic Technologies / International Society for Ceramics in Medicine / International Society for Magnetic Resonance in Medicine / International Society for Optical Engineering / International Society for Structural Health Monitoring of Intelligent Infrastructure / International Society of Nanotechnology / International Union of Crystallography / Materials Information Society / Minerals, Metals & Materials Society / Minerals, Metals & Materials Society of AIME / Optical Society of America / Society for Advancement of Material and Process Engineering / Society for Biomaterials / Society for Experimental Mechanics / Society for Information Display / Society for Mining, Metallurgy & Exploration / Society for Photo-Optical Instrumentation Engineers / Society for Rheology / Society for the Advancement of Material and Process Engineering / Society of Automotive Engineers / Society of Cosmetic Chemists / Society of Glass Technology / Society of Manufacturing Engineers / Society of Materials Science / Society of Naval Architects and Marine Engineers / Society of Plastics Engineers / Society of Tribologists and Lubrication Engineers / The Minerals, Metals & Materials Society
Smart Material Societies in Europe:
Advanced Porous Materials Association / Association of German Dental Manufacturers / Association of Steel-Concrete Composite Structures / Branch Association Polymers / Composites UK / Construction Products Association / Craft Potters Association / Craft Potters Association of Great Britain / European Photovoltaic Industry Association / Oxford University Materials Society / European Plastics Converters / Polish Materials Science Society / Polish Society for Biomaterials / European Ceramic Society / Society of Chemical Industry / European Society for Biomaterials / Wey Ceramics Society / Italian Association for Metallurgy / London Materials Society / The Institute of Materials, Minerals & Mining / Materials Societies Oxford Materials / Royal Society of Chemistry / European Materials Research Society / The Oriental Ceramic Society / Tiles & Architectural Ceramics Society / United Kingdom Society for Biomaterials / Energy Materials Group / European Materials Research Society / The Federation of European Materials Societies / Society for Biomaterials / Scandinavian Society for Biomaterials / European Society for Composite Materials / The Polymer Society / German Engineering Materials Science Centre / Swiss Federal Laboratories for Materials Science and Technology / European Nanoscience and Nanotechnology Association / Nanotechnology Industries Association / European Nanotechnology Trade Association / The Federation of European Materials / The European Ceramic Industry Association / The European Composites Industry Association / European Association for ETICS / European Plastics Distributors Association / Polymer Associations / New German Plastics Federation / European Bioplastics / Composites Germany / German Association of Nanotechnology / The British Plastics Federation / Polymer Machinery Manufacturers and Distributors Association / The Telford Polymer Association / Rubber and plastic trade associations / Scottish Plastics and Rubber Association / Rubber and Plastic Research Association / Bio-based and Biodegradable Industries Association / Ceramic Association of La Borne / Association of Advanced Ceramics / French Manufacturers / Associations of Ceramics Cities / Morbi Ceramic Association / Nanotechnology Industries Association / European Plastics Distributors Association / Plastics Industry Association / Trade Associations / Flexible Packaging Association / French Chinese Association of Energy / Fuel Cell and Hydrogen Energy Association / German Association of Energy and Water / German Association of the Automotive Industry / German Energy Storage Association German Solar Industry Association / German Solar Association / Helmholtz Association of German Research Centers / International Association for Energy Economics / Italian Industry Association for Composite Materials / Natural Materials Association board / Northern Potters Association / Spanish Association of Cities of Ceramics / Spanish Association of Plastics Industry / Spanish Ceramic Tile Manufacturers Association / Spanish Renewable Energy Association / The British Composites Society / The Energy Materials Industrial Research Initiative Association / The German Building Materials Association / UK Pottery Associations / Westcountry Potters Association / The German Ceramic Society
Smart Material Societies in Asia:
Chinese Ceramic Society / The Minerals, Metals & Materials Society / Japan Society for Composite Materials / Japan Society for Precision Engineering / Japan Society for Technology of Plasticity / Japan Society for the Promotion of Science / Japan Society of Applied Physics / Chinese Materials Research Society / Indian Ceramic Society / Indian Institute of Ceramics / Indian Institute of Metals / Indian Materials Congress / Indian Rubber Manufacturers Research Association / Indian Society for Non-Destructive Testing / Indian Society for Surface Science and Technology / Institute of Materials Malaysia / Iranian Association of Foundrymen / Iranian Association of Metallurgical Engineers / Iranian Association of Welding / Chinese Society for Biomaterials / Chinese Society for Composite Materials / Chinese Society for Corrosion and Protection / Chinese Society for Metals / Indian Association of Crystal Growth / Iranian Ceramic Society / Iranian Chemical Society / Iranian Metallurgical Society / Iranian Polymer Society / Iranian Rubber Industries Association / Iranian Society for Composite Materials / Iranian Society of Corrosion / Iranian Society of Crystallography / Iranian Society of Electrochemistry / Iranian Society of Glass and Ceramics / Iranian Society of Materials Science and Engineering / Iranian Society of Nanotechnology / Iranian Society of Rheology / Japan Institute of Ceramics / Japan Institute of Metals / Japan Society of Biomaterials / Japan Society of Materials Science / Japan Society of Powder and Powder Metallurgy / Japanese Society of Engineering Education / Korea Society of Nanoscience and Nanotechnology / Korean Ceramic Society / Korean Institute of Metals and Materials / Korean Society for Composite Materials / Korean Society of Materials Science / Korean Society of Mechanical Engineers / Materials Research Society of Malaysia / Pakistan Ceramic Society / Materials Science and Technology Society of Japan / Pakistan Institute of Engineering and Applied Sciences / Materials Research Society of Pakistan / Materials Research Society of Singapore / Materials Research Society of Thailand / Materials Research Society of the Philippines / Materials Research Society of Vietnam / Materials Science and Engineering Society of India / Materials Science and Technology Society, India / Materials Science Society of Bangladesh / Materials Science Society of Japan / Materials Science Society of Thailand / Materials Research Society of Bangladesh / Materials Research Society of Hong Kong / Materials Research Society of India / Materials Research Society of Indonesia / Materials Research Society of Iran / Materials Research Society of Japan / Materials Research Society of Korea / Pakistan Institute of Engineering and Technology / Pakistan Institute of Materials Science / Pakistan Institute of Physics / Pakistan Society of Chemical Engineers / Pakistan Society of Nanoscience and Nanotechnology / Philippine Institute of Chemical Engineers / Philippine Society of Nanotechnology / Singapore Institute of Materials Management / Singapore Society of Materials Science / Society of Materials Science, Japan / Society of Materials Science, Taiwan / Society of Plastic Engineers, India / Society of Plastic Engineers, Korea / Society of Plastics Engineers, Japan / Taiwan Ceramic Society / Taiwan Institute of Chemical Engineers / Taiwan Rubber Science and Technology Association / Taiwan Society for Biomaterials / Taiwan Society for Biomaterials and Controlled Release / Taiwan Society for Composite Materials / Taiwan Society for Engineering Education / Taiwan Society of Coatings Technology / Taiwan Society of Composite Materials / Taiwan Society of Nanotechnology / Thai Ceramic Society
Smart Materials Courses:
Introduction to Smart Materials / Fundamentals of Materials Science and Engineering / Advanced Materials for Engineering Applications / Nanomaterials and Nanotechnology / Shape Memory Materials / Piezoelectric Materials / Thermoelectric Materials / Electroactive Polymers / Self-Healing Materials / Biomimetic Materials / Stimuli-Responsive Materials / Smart Textiles and Wearable Technology / Functional Materials in Electronics / Advanced Composites / Quantum Dots and Their Applications / Liquid Crystal Materials / Metamaterials / Photonic Crystals / Magnetic Smart Materials / Ferroelectric Materials / Hydrogels and Soft Robotics / Responsive Surfaces and Coatings / Bio-inspired Materials / Smart Concrete and Infrastructure Materials / Smart Coatings for Corrosion Protection / Smart Packaging Materials / 3D Printing of Smart Materials / Smart Sensors and Actuators / Smart Drug Delivery Systems / Smart Hydrogels for Biomedical Applications / Smart Membranes for Water Treatment / Smart Polymers in Biotechnology / Functional Nanocomposites / Smart Energy Materials / Solar Cells and Photovoltaics / Thermochromic Materials / Conductive Polymers / Advanced Ceramics / Microfluidics and Lab-on-a-Chip Devices / Bioactive Glasses and Ceramics / Bioelectronics and Bio-Interfaces / Smart Surfaces for Anti-fouling Applications / Responsive Materials in Microfluidics / Flexible Electronics and Displays / Smart Packaging for Food Preservation / Smart Windows and Glazing / Shape-changing Materials / Responsive Polymers for Drug Delivery / Magnetic Nanoparticles in Biomedicine / Smart Fabrics for Healthcare Monitoring / Stimuli-Responsive Nanoparticles / Advanced Semiconductor Materials / BioMEMS and Bio-NEMS / Electrochromic Materials / Piezoelectric Energy / Harvesting / Smart Agriculture Materials / Self-cleaning Surfaces and Materials / Magnetic Levitation Systems / Smart Adhesives and Sealants / Biohybrid Materials / Stimuli-Responsive Drug Release Systems / Smart Surfaces for Anti-icing Applications / Smart Glasses and Eyewear / Electrically Conductive Hydrogels / Smart Bandages and Wound Dressings / Flexible Energy Storage Devices / Shape Memory Alloys in Biomedicine / Responsive Hydrogels for Tissue Engineering / Smart Coatings for Anti-fogging Applications / Biomimetic Sensors and Actuators / Smart Inks and Printing Technologies / Responsive Microgels for Drug Delivery / Smart Dust and Wireless Sensor Networks / Self-assembling Materials / Smart Fabrics for Environmental Monitoring / Bio-inspired Robotics Materials / Responsive Fibers and Fabrics / Smart Nanoparticles for Cancer Therapy / Autonomous Materials / Smart Implants and Prosthetics / Responsive Inks for 4D Printing / Smart Geotextiles for Civil Engineering / Responsive Filters and Separators / Self-healing Concrete / Smart Fabrics for Sports and Fitness / Responsive Lubricants and Coatings / Smart Surfaces for Water Harvesting / Bio-inspired Soft Robotics / Responsive Coatings for Structural Health Monitoring / Smart Grid Materials / Stimuli-Responsive Hydrocolloids / Self-cleaning Fabrics and Textiles / Smart Building Materials / Responsive Gels for SoftRobotics / Smart Insecticides and Pest Control Materials / Bio-inspired Adhesives / Responsive Fluids for Microfluidic Devices / Smart Inks for Electronic Skin Applications
Top 100 Smart Materials Start-ups:
GraphenTech - Spain / NanoSteel - United States / InMat - United States / Ossila - United Kingdom / GelSight - United States / Nanotech Energy - United States / OxMet Technologies - United Kingdom / 4D Heat - Israel / MetaMateria - United States / Nanocomp Technologies - United States / LiquiGlide - United States / QD Laser - Japan / Twisted Light - Australia / Nanowear - United States / Hummingbird Kinetics - Canada / Cerion Nanomaterials - United States / AMO GmbH - Germany / Strain Solutions - United States / Nanotech Biomachines - Israel / NanoXplore - Canada / Carbice Corporation - United States / Versarien - United Kingdom / Angstron Materials - United States / Nanoscale Corporation - United States / Nanotech Security - Canada / Sila Nanotechnologies - United States / Grolltex - United States / BlueDot Photonics - United States / Graphmatech - Sweden / Xolve - United States / Peratech - United Kingdom / Adama Innovations - United States / Neutron Therapeutics - United States / Wearality Corporation - United States / Inpria - United States / Crystal IS - United States / NBD Nanotechnologies - United States / Gauzy - Israel / Vorbeck Materials - United States / Lux Research - United States / Nantero - United States / Aerogel Technologies - United States / Lumiphore - United States / NanoMech - United States / Alchemy Materials - United States / BrightVolt - United States / Novomer - United States / Mosaic Materials - United States / Nanocomposix - United States / Airgain - United States / WattGlass - United States / Hyperion Technologies - United States / 6K - United States / Altered Silicon - United States / Cambrios Technologies - United States / P2i - United Kingdom / Altierre - United States / SupraG Energy - United States / Blue Current - United States / Tethon 3D - United States / Front Edge Technology - United States / BluGlass - Australia / Spotnails - United States / Novasentis - United States / Echion Technologies - United Kingdom / SoftWear Automation - United States / SecureRF - United States / Vartega - United States / GEn1E Lifesciences - United States / Immersion Corporation - United States / EnSol AS - Norway / ZAGENO - Germany / Liquid Wire - United States / Optitune - Finland / Oxonica - United Kingdom / Strayos - United States / Neurable - United States / Adhesys Medical - Germany / GigaSolar Materials Corp. - Taiwan / Embr Labs - United States / Eneida Wireless - Portugal / Hypersciences - United States / Modern Meadow - United States / Solugen - United States / Atomic X - Canada / CarbonCure Technologies - Canada / Origin Materials - United States / Vivonics - United States / Polysync Technologies - United States / Invisage Technologies - United States / Echogen Power Systems - United States / Carbon3D - United States / Nitride Solutions - United States / LabGenius - United Kingdom / Clear Blue Technologies - Canada / Solantro Semiconductor - Canada / Smart Wires - United States / Solstice Energy Solutions - United States / Enevate Corporation - United States
Smart Materials University in Europe:
University of Cambridge / Imperial College London / University of Oxford / ETH Zurich / Technical University of Munich / University of Groningen / KU Leuven / Technical University of Denmark / RWTH Aachen University / University of Helsinki / University of Manchester / University College London / University of Edinburgh / University of Paris-Saclay / University of Twente / University of Stuttgart / University of Zurich / University of Warsaw / TU Delft / Ecole Polytechnique Federale de Lausanne / University of Liverpool / University of Bristol / Technical University of Berlin / University of Southampton / University of Copenhagen / University of Leuven / University of Glasgow / Karlsruhe Institute of Technology / University of Sheffield / University of Strasbourg / Aalto University / University of Birmingham / University of Nottingham / TU Wien / University of Oslo / University of Exeter / University of Vienna / University of Cologne / University of Southampton / University of Aveiro / École Polytechnique / University of Freiburg / University of Lorraine / University of Patras / University of Seville / University of Bologna / University of Porto / University of Zagreb / University of Rennes
Smart Materials University in USA:
Massachusetts Institute of Technology / Stanford University / University of California / California Institute of Technology / Harvard University / Georgia Institute of Technology / University of Illinois at Urbana-Champaign / Northwestern University / University of Michigan / University of Pennsylvania / Cornell University / Purdue University / University of Texas at Austin / Carnegie Mellon University / University of California, Los Angeles / University of Wisconsin-Madison / University of Minnesota Twin Cities / University of Washington / Duke University / University of Florida / Texas A&M University / Columbia University / University of Maryland / University of Virginia / University of Colorado Boulder / University of California, Irvine / Ohio State University / North Carolina State University - Raleigh / Virginia Tech - Blacksburg / University of Arizona - Tucson / University of Delaware
Smart Materials University in Asia:
Tsinghua University / National University of Singapore / Seoul National University / Tokyo Institute of Technology / Indian Institute of Technology / Pohang University of Science and Technology / Shanghai Jiao Tong University /
Nanyang Technological University / University of Tokyo / KAIST-Korea Advanced Institute of Science and Technology / Hong Kong University of Science and Technology / National Taiwan University / Zhejiang University / Indian Institute of Science / Tohoku University / King Abdullah University of Science and Technology / National Tsing Hua University / Osaka University / ShanghaiTech University / Peking University / Nankai University / Indian Institute of Technology / National Chiao Tung University / Hanyang University / University of Seoul / Hong Kong Polytechnic University / Tokyo Metropolitan University / Indian Institute of Technology / National Cheng Kung University / National University of Science and Technology / Huazhong University of Science and Technology / Indian Institute of Technology / Kyoto University / Beijing Institute of Technology / National Central University / Kyushu University / University of Malaya / Chulalongkorn University / National University of Sciences and Technology / Beijing University of Chemical Technology / Indian Institute of Technology (IIT) Roorkee / National Yang Ming Chiao Tung University / Hefei University of Technology / Bangladesh University of Engineering and Technology / Sharif University of Technology / National Dong Hwa University
Smart Materials University in Middle-East:
King Abdullah University of Science and Technology / American University of Beirut / Qatar University / King Fahd University of Petroleum and Minerals / Sharif University of Technology / Technion - Israel Institute of Technology / United Arab Emirates University / King Saud University / Tehran University of Medical Sciences / Isfahan University of Technology / King Abdulaziz University / Iran University of Science and Technology / Ben-Gurion University of the Negev / University of Tehran / Jordan University of Science and Technology / Birzeit University / King Khalid University / Sultan Qaboos University / University of Jordan / University of Haifa / Alexandria University / IstanbulTechnical University / Lebanese American University / University of Tabriz / Kuwait University / Al-Balqa' Applied University / Istanbul University / University of Sulaimani / Jordan University of Technology / Eastern Mediterranean University / University of Baghdad / Istanbul Medeniyet University / University of Tehran / King Abdulaziz City for Science and Technology / Istanbul Aydin University / Shahid Beheshti University / Istanbul University-Cerrahpasa / Al-Nahrain University / Yildiz Technical University / Isik University
Contact us now and we will make your event unique & unforgettable
All numbers indicates percentage %
Europe
North America
Middle East
Asia Pacific
Africa
All numbers indicates percentage %
Material Scientists, Engineers, and Researchers
Energy Sector Professionals
Structural Engineers and Architects
Academics and Educators
Students and Early Career Researchers
Industry Representatives
Manufacturing Companies
Investors and Entrepreneurs